Структурная организация и функционирование АТР-зависимой Lon-протеиназы из Archaeoglobus fulgidus
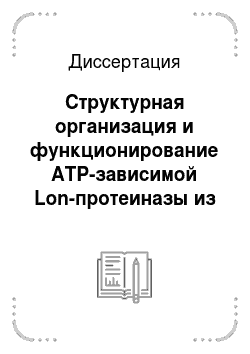
Диссертация
Механизмы деструкции белков играют ключевую роль в поддержании сохранности клеточного протеома, обеспечивая удаление нежелательных или опасных белков, а также быструю и адекватную регуляцию биологического ответа на изменяющиеся условия. Деградацию белков в клетках бактерий, эукариот и архебактерий осуществляют АТР-зависимые протеиназы, функционирующие наряду с другими известными ААА±АТР-азами… Читать ещё >
Содержание
- Глава 1. РЕГУЛЯТОРНЫЕ ПРОТЕИНАЗЫ И ПРОТЕОЛИТИЧЕСКИЕ СИСТЕМЫ В АРХЕЯХ (Обзор литературы)
- 1. Энергозависимый селективный протеолиз — механизм поддержания внутриклеточного протеома. Известные к настоящему времени энергозависимые пептидгидролазы
- 1. 1. Характерные особенности, А ТР-азных составляющих AAA*-протеиназ
- 1. 1. 1. Общая характеристика AAA*-белков
- 1. 1. 2. А ТР-азные компоненты ААА±протеиназ
- 1. 1. 2. 1. Экстра-домены и белки-адаптеры A TP-зависимых протеиназ
- 1. 1. 2. 2. Нуклеотидсвязывающие и а-спирализованные домены АТР-зависимых протеиназ
- 1. 1. Характерные особенности, А ТР-азных составляющих AAA*-протеиназ
- 1. 2. Протеолитические составляющие энергозависимых протеиназ
- 1. 3. Функционирование, А TP-зависимых протеиназ и протеолитических комплексов
- 1. Энергозависимый селективный протеолиз — механизм поддержания внутриклеточного протеома. Известные к настоящему времени энергозависимые пептидгидролазы
- 2. Протеиназы и протеолитические системы архебактерий
- 2. 1. Общая характеристика пула протеиназ архей 35 2.1.1. А TP-независимые протеиназы гипертермофильных микроорганизмов
- 2. 2. Регуляторные протеиназы архей
- 2. 2. 1. А TP-зависимые протеиназы архей
- 2. 2. 2. Интегральные мембранные протеиназы
Список литературы
- Goldberg A.L. (1992) The mechanism and functions of ATP-dependent proteases in bacterial and animal cells. Eur. J. Biochem., 203(1−2), 9−23.
- Pahl H.L., Baeuerle P.A.(1996) Control of gene expression by proteolysis. Curr. Opin. Cell Biol., 8(3), 340−7.
- Martoglio B. (1999) Transmembrane segment proteases. Protoplasma, 207,141−146.
- Gottesman S. (1999) Regulation by proteolysis: developmental switches. Curr. Opin. Microbiol., 2(2), 142−7.
- King R.W., Deshaies R.J., Peters J.M., Kirschner M.W. (1996) How proteolysis drives the cell cycle. Science., 274(5293), 1652−9
- Gottesman S. (2003) Proteolysis in bacterial regulatory circuits. Annu Rev. Cell Dev. Biol, 19, 565−87
- Sullivan J.A., Shirasu K, Deng XW (2003) Nat. Rev. Genet., 4(12), 948−58.
- Rothschild L.J., Mancinelli R.L. (2001) Life in extreme environments. Nature, 409(6823), 1092−101.
- Ward D. E, Shockley K.R., Chang L.S., Levy R. D, Michel J.K., Conners S.B., Kelly R.M. (2002) Proteolysis in hyperthermophilic microorganisms. Archaea, 1(1), 63−74.
- Coux O., Tanaka K., Goldberg A.L. (1996) Structure and functions of the 20S and 26S proteasomes. Annu. Rev. Biochem., 65, 801−47.
- Maupin-Furlow J.A., Wilson H.L., Kaczowka S.J., Ou M.S. (2000) Proteasomes in the archaea: from structure to function. Front. Biosci., 5, 837−65.
- Erdmann R., Wiebel F.F., Flessau A., Rytka J., Beyer A., Frohlich K.U., Kunau W.H. (1991) PAS1, a yeast gene required for peroxisome biogenesis, encodes a member of a novel family of putative ATPases. Cell, 64(3), 499−510.
- Iyer L.M., Leipe D.D., Koonin E.V., Aravind L. (2004) Evolutionary history and higher order classification of AAA+ ATPases. J. Struct. Biol., 146(1−2), 11−31.
- Confalonieri F., Duguet M. (1995) A 200-amino acid ATPase module in search of a basic function. Bioessays, 17(7), 639−650.
- Beyer A. (1997) Sequence analysis of the AAA protein family. Protein Sci., 6(10), 20 432 058.
- Patel S., Latterich M. (1998) The AAA team: related ATPases with diverse functions. Trends Cell. Biol., 8(2), 65−71.
- Lupas A.N., Martin J. (2002) AAA proteins. Curr. Opin. Struct. Biol., 12(6), 746−753.
- Neuwald A.F., Aravind L., Spouge J.L., Koonin E.V. (1999) AAA+: A class of chaperone-like ATPases associated with the assembly, operation, and disassembly of protein complexes. Genome Res., 9(1), 27−43.
- Frickey Т., Lupas A.N. (2004) Phylogenetic analysis of AAA proteins. J. Struct. Biol., 146(1−2), 2−10.
- Hersch GL, Burton RE, Bolon DN, Baker ТА, Sauer RT. (2005) Asymmetric interactions of ATP with the AAA+ ClpX6 unfoldase: allosteric control of a protein machine. Cell, 121(7), 1017−27.
- Smith C.K., Baker T.A., Sauer R.T. (1999) Lon and Clp family proteases and chaperones share homologous substrate-recognition domains. Proc. Natl. Acad. Sci. USA, 96(12), 66 786 682.
- Schirmer E.C., Glover J.R., Singer M.A., Lindquist S. (1996) HSPlOO/Clp proteins: a common mechanism explains diverse functions. Trends Biochem Sci., 21(8), 289−96.
- Singh SK, Rozycki J, Ortega J, Ishikawa T, Lo J, Steven AC, Maurizi MR. (2001) Functional domains of the ClpA and ClpX molecular chaperones identified by limited proteolysis and deletion analysis. J Biol Chem., 276(31), 29 420−9.
- Hattendorf D.A., Lindquist S.L. (2002) Analysis of the AAA sensor-2 motif in the C-terminal ATPase domain of Hspl04 with a site-specific fluorescent probe of nucleotide binding. Proc. Natl. Acad. Sci. USA, 99(5), 2732−2737.
- Dougan D.A., Mogk A., Zeth K., Turgay K" Bukau B. (2002) AAA+ proteins and substrate recognition, it all depends on their partner in crime. FEBS Lett., 529(1), 6−10.
- Ahmadian M.R., Stege P., Scheffzek K., Wittinghofer A. (1997) Confirmation of the arginine-finger hypothesis for the GAP-stimulated GTP-hydrolysis reaction of Ras. Nat. Struct. Biol., 4(9), 686−689.
- Hattendorf D.A., Lindquist S.L. (2002) Cooperative kinetics of both Hspl04 ATPase domains and interdomain communication revealed by AAA sensor-1 mutants. EMBO J., 21(1−2), 1221.
- Tomoyasu Т., Mogk A., Langen H., Goloubinoff P., Bukau B. (2001) Genetic dissection of the roles of chaperones and proteases in protein folding and degradation in the Escherichia coli cytosol. Mol. Microbiol., 40(2), 397−413.
- Keiler K.C., Waller P.R., Sauer R.T. (1996) Role of a peptide tagging system in degradation of proteins synthesized from damaged messenger RNA. Science, 271(5251), 990−993.
- Martin J., Gruber M., Lupas A.N. (2004) Coiled coils meet the chaperone world. Trends Biochem. Sci., 29(9), 455−458.
- Mogk A., Dougan D., Weibezahn J., Schlieker C., Turgay K., Bukau B. (2004) Broad yet high substrate specificity: the challenge of AAA+ proteins. J. Struct. Biol., 146(1−2), 90−98.
- Rouiller I., DeLaBarre В., May A.P., Weis W.I., Brunger A.T., Milligan R.A., Wilson-Kubalek E.M. (2002) Conformational changes of the multifunction p97 AAA ATPase during its ATPase cycle. Nat. Struct. Biol., 9(12), 950−957.
- Vale R.D. (2000) AAA proteins. Lords of the ring. J. Cell. Biol., 150(1), F13−19.
- Dougan D.A., Mogk A., Bukau B. (2002) Protein folding and degradation in bacteria: to degrade or not to degrade? That is the question. Cell. Mol. Life Sci., 59(10), 1607−1616.
- Voges D, Zwickl P, Baumeister W. (1999) The 26S proteasome: a molecular machine designed for controlled proteolysis. Annu. Rev. Biochem., 68, 1015−68.
- Singh S.K., Grimaud R., Hoskins J.R., Wickner S., Maurizi M.R. (2000) Unfolding and internalization of proteins by the ATP-dependent proteases CIpXP and ClpAP. Proc. Natl. Acad. Sci. USA, 97(16), 8898−8903.
- Kim Y.I., Burton R.E., Burton B.M., Sauer R.T., Baker T.A. (2000) Dynamics of substrate denaturation and translocation by the CIpXP degradation machine. Mol. Cell, 5(4), 639−648.
- Weber-Ban E.U., Reid B.G., Miranker A.D., Horwich A.L. (1999) Global unfolding of a substrate protein by the HsplOO chaperone ClpA. Nature, 401(6748), 90−93.
- Grimaud R., Kessel M., Beuron F., Steven A.C., Maurizi M.R. (1998) Enzymatic and structural similarities between the Escherichia coli ATP-dependent proteases, CIpXP and ClpAP. J. Biol Chem., 273(20), 12 476−12 481.
- Singh S.K., Maurizi M.R. (1994) Mutational analysis demonstrates different functional roles for the two ATP-binding sites in ClpAP protease from Escherichia coli. J. Biol. Chem., 269(47), 29 537−29 545.
- Seol J.H., Yoo S.J., Shin D.H., Shim Y.K., Kang M.S., Goldberg A.L., Chung C.H. (1997) The heat-shock protein HslVU from Escherichia coli is a protein-activated ATPase as well as an ATP-dependent proteinase. Eur. J. Biochem., 247(3), 1143−50.
- Kanemori M., Yanagi H., Yura T. (1999) The ATP-dependent HslVU/ClpQY protease participates in turnover of cell division inhibitor SulA in Escherichia coli. J. Bacteriol., 181(12), 3674−3680.
- Roudiak S.G., Shrader Т.Е. (1998) Functional role of the N-terminal region of the Lon protease from Mycobacterium smegmatis. Biochemistry, 37(32), 11 255−11 263.
- Ebel W., Skinner M.M., Dierksen K.P., Scott J.M., Trempy J.E. (1999) A conserved domain in Escherichia coli Lon protease is involved in substrate discriminator activity. J. Bacteriol., 181(7), 2236−2243.
- Kuroda A., Nomura K., Ohtomo R., Kato J., Ikeda Т., Takiguchi N., Ohtake H., Kornberg A. (2001) Role of inorganic polyphosphate in promoting ribosomal protein degradation by the Lon protease in E. coli. Science, 293(5530), 705−708.
- Lo J.H., Baker T.A., Sauer R.T. (2001) Characterization of the N-terminal repeat domain of Escherichia coli CIpA-A class I Clp/HSP 100 ATPase. Protein Sci., 10(3), 551 -9.
- Hinnerwisch J., Reid B.G., Fenton W.A., Horwich A.L. (2005) Roles of the N-domains of the ClpA unfoldase in binding substrate proteins and in stable complex formation with the ClpP protease. J. Biol. Chem., 280(49), 40 838−44.
- Dougan D.A., Reid B.G., Horwich A.L., Bukau B. (2002) CIpS, a substrate modulator of the ClpAP machine. Mol. Cell, 9(3), 673−683.
- Zeth K., Ravelli R.B., Paal K., Cusack S., Bukau В., Dougan D.A. (2002) Structural analysis of the adaptor protein CIpS in complex with the N-terminal domain of ClpA. Nat. Struct. Biol., 9(12), 906−911.
- Banecki В., Wawrzynow A., Puzewicz J., Georgopoulos C., Zylicz M. (2001) Structure-function analysis of the zinc-binding region of the ClpX molecular chaperone. J. Biol. Chem., 276(22), 18 843−18 848.
- Wojtyra U.A., Thibault G., Tuite A., Houry W.A. (2003) The N-terminal zinc binding domain of ClpX is a dimerization domain that modulates the chaperone function. J. Biol. Chem., 278(49), 48 981−48 990.
- Flynn J.M., Neher S.B., Kim Y.I., Sauer R.T., Baker T.A. (2003) Proteomic discovery of cellular substrates of the ClpXP protease reveals five classes of ClpX-recognition signals. Mol. Cell, 11(3), 671−683.
- Levchenko I., Seidel M., Sauer R.T., Baker T.A. (2000) A specificity-enhancing factor for the ClpXP degradation machine. Science, 289(5488), 2354−2356.
- Wah D.A., Levchenko I., Baker T.A., Sauer R.T. (2002) Characterization of a specificity factor for an AAA+ ATPase: assembly of SspB dimers with ssrA-tagged proteins and the ClpX hexamer. Chem. Biol., 9(11), 1237−1245.
- Wah D.A., Levchenko I., Rieckhof G.E., Bolon D.N., Baker T.A., Sauer R.T. (2003) Flexible linkers leash the substrate binding domain of SspB to a peptide module that stabilizes delivery complexes with the AAA+ ClpXP protease. Mol. Cell, 12(2), 355−363.
- Dougan D.A., Weber-Ban E., Bukau B. (2003) Targeted delivery of an ssrA-tagged substrate by the adaptor protein SspB to its cognate AAA+ protein ClpX. Mol. Cell, 12(2), 373−380.
- Song H.K., Eck M.J. (2003) Structural basis of degradation signal recognition by SspB, a specificity-enhancing factor for the ClpXP proteolytic machine. Mol. Cell, 12(1), 75−86.
- Muffler A., Fischer D., Altuvia S., Storz G., Hengge-Aronis R. (1996) The response regulator RssB controls stability of the sigma (S) subunit of RNA polymerase in Escherichia coli. EMBOJ., 15(6), 1333−1339.
- Zhou Y., Gottesman S., Hoskins J.R., Maurizi M.R., Wickner S. (2001) The RssB response regulator directly targets sigma (S) for degradation by ClpXP. Genes Dev., 15(5), 627−637.
- Tang M., Shen X., Frank E.G., O’Donnell M., Woodgate R., Goodman M.F. (1999) UmuD'(2)C is an error-prone DNA polymerase, Escherichia coli pol V. Proc. Natl. Acad. Sci. USA, 96(16), 8919−8924.
- Gonzalez M., Rasulova F., Maurizi M.R., Woodgate R. (2000) Subunit-specific degradation of the UmuD/D' heterodimer by the ClpXP protease: the role of trans recognition in UmuD' stability. EMBOJ., 19(19), 5251−5258.
- Ito K., Akiyama Y. (2005) Cellular functions, mechanism of action, and regulation of FtsH protease. Annu. Rev. Microbiol., 59,211−231.
- Kihara A., Akiyama Y., Ito K. (1996) A protease complex in the Escherichia coli plasma membrane: HflKC (HflA) forms a complex with FtsH (HflB), regulating its proteolytic activity against SecY. EMBOJ., 15(22), 6122−6131.
- Saikawa N., Akiyama Y., Ito K. (2004) FtsH exists as an exceptionally large complex containing HflKC in the plasma membrane of Escherichia coli. J. Struct. Biol., 146(1−2), 123 129.
- Akiyama Y., Kihara A., Mori H., Ogura Т., Ito K. (1998) Roles of the periplasmic domain of Escherichia coli FtsH (HflB) in protein interactions and activity modulation. J. Biol. Chem., 273(35), 22 326−22 333.
- Akiyama Y., Ito K. (2000) Roles of multimerization and membrane association in the proteolytic functions of FtsH (HflB). EMBOJ, 19(15), 3888−95.
- Akiyama Y., Ito K. (2001) Roles of homooligomerization and membrane association in ATPase and proteolytic activities of FtsH in vitro. Biochemistry, 40(25), 7687−93.
- Bieniossek С., Schalch Т., Bumann M., Meister M., Meier R., Baumann U. (2006) The molecular architecture of the metalloprotease FtsH. Proc. Natl. Acad. Sci. USA., 103(9), 306 671.
- Song H.K., Hartmann C., Ramachandran R., Bochtler M., Behrendt R., Moroder L., Huber R. (2000) Mutational studies on HslU and its docking mode with HslV. Proc. Natl. Acad. Sci. USA, 97(26), 14 103−14 108.
- Kwon A.R., Kessler B.M., Overkleeft H.S., McKay D.B. (2003) Structure and reactivity of an asymmetric complex between HslV and I-domain deleted HslU, a prokaryotic homolog of the eukaryotic proteasome. J. Mol. Biol., 330(2), 185−195.
- Kim Y.I., Levchenko I., Fraczkowska K., Woodruff R.V., Sauer R.T., Baker T.A. (2001) Molecular determinants of complex formation between Clp/HsplOO ATPases and the ClpP peptidase. Nat. Struct. Biol., 8(3), 230−233.
- Sousa M.C., Trame C.B., Tsuruta H., Wilbanks S.M., Reddy V.S., McKay D.B. (2000) Crystal and solution structures of an HslUV protease-chaperone complex. Cell, 103(4), 633 643.
- Guo F" Maurizi M.R., Esser L., Xia D. (2002) Crystal structure of ClpA, an HsplOO chaperone and regulator of ClpAP protease. J. Biol. Chem., 277(48), 46 743−46 752.
- Kim DY, Kim KK (2003) Crystal structure of ClpX molecular chaperone from Helicobacter pylori. J. Biol. Chem., 278(50), 50 664−50 670.
- Bochtler M., Hartmann C" Song H.K., Bourenkov G.P., Bartunik H.D., Huber R. (2000) The structures of HslU and the ATP-dependent protease HsIU-HsIV. Nature, 403(6771), 800−805.
- Trame C.B., McKay D.B. (2001) Structure of Haemophilus influenzae HslU protein in crystals with one-dimensional disorder twinning. Acta Crystallogr. D. Biol. Crystallogr., 57(Pt 8), 1079−90.
- Sousa M.C., Kessler B.M., Overkleeft H.S., McKay D.B. (2002) Crystal structure of HslUV complexed with a vinyl sulfone inhibitor: corroboration of a proposed mechanism of allosteric activation of HslV by HslU. J. Mol. Biol., 318(3), 779−785.
- Krzywda S., Brzozowski A.M., Verma C., Karata K., Ogura Т., Wilkinson A.J. (2002) The crystal structure of the AAA domain of the ATP-dependent protease FtsH of Escherichia coli at 1.5 A resolution. Structure (Camb), 10(8), 1073−1083.
- Niwa H., Tsuchiya D., Makyio H., Yoshida M., Morikawa K. (2002) Hexameric ring structure of the ATPase domain of the membrane-integrated metalloprotease FtsH from Thermus thermophilus HB8. Structure (Camb), 10(10), 1415−1423.
- Barrett A.J., Rawlings N.D., O’Brien E.A. (2001) The MEROPS database as a protease information system. J. Struct. Biol., 134(2−3), 95−102.
- Goldschmidt R. (1970) In vivo degradation of nonsense fragments in E. coli. Nature, 228(277), 1151−1154.
- Chung C.H., Goldberg A.L. (1981) The product of the lon (capR) gene in Escherichia coli is the ATP-dependent protease, protease La. Proc. Natl. Acad. Sci. USA, 78(8), 4931−4935.
- Charette M.F., Henderson G.W., Markovitz A. (1981) ATP hydrolysis-dependent protease activity of the lon (capR) protein of Escherichia coli K-12. Proc. Natl. Acad. Sci. USA, 78(8), 4728−4732.
- Америк А.Ю., Чистякова Л. Г., Остроумова Н. И., Гуревич А. И., Антонов В. К. (1988) Клонирование, экспрессия и структура функционально активного укороченного гена lon Escherichia coli. Биоорган, химия, 14(3), 408−411.
- Америк А.Ю., Антонов В. К., Остроумова Н. И., Ротанова Т. В., Чистякова Л. Г. (1990) Клонирование, структура и экспрессия полноразмерного гена lon Escherichia coli, кодирующего АТР-зависимую Ьа-протеиназу. Биоорган, химия, 16(7), 869−880.
- Amerik A.Yu., Antonov V.K., Gorbalenya A.E., Kotova S.A., Rotanova T.V., Shimbarevich E.V. (1991) Site-directed mutagenesis of La protease. A catalytically active serine residue. FEBSLett., 287(1−2), 211−214.
- Ротанова T.B. (1999) Структурно-функциональные особенности АТР-зависимой Lon-протеиназы из Escherichia coli. Биоорган, химия, 25(12), 883−891.
- Starkova N.N., Koroleva E.P., Rumsh L.D., Ginodman L.M., Rotanova T.V. (1998) Mutations in the proteolytic domain of Escherichia coli protease Lon impair the ATPase activity of the enzyme. FEBSLett., 422(2), 218−220.
- Ротанова T.B., Мельников Э. Э., Цирульников К. Б. (2003) Каталитическая диада Ser -Lys в активном центре АТР-зависимой Lon-протеиназы Escherichia coli. Биоорган, химия, 29(1), 97−99.
- Chung, C.H., Goldberg, A.L. (2004) Endopeptidase La. In Handbook of Proteolytic Enzymes, 2 edn (Barrett, A. J., Rawlings.N.D. and Woessner, J.F. eds), 1998−2002.
- Nishii W., Maruyama Т., Matsuoka R., Muramatsu T. and Takahashi, K. (2002) The unique sites in SulA protein preferentially cleaved by ATP-dependent Lon protease from Escherichia coli. Eur. J. Biochem., 269,451−457.
- Waxman L., Goldberg A.L. (1985) Protease La, the lon gene product, cleaves specific fluorogenic peptides in an ATP-dependent reaction. J. Biol. Chem., 260(22), 12 022−12 028.
- Thomas-Wohlever, J., Lee, I. (2002) Kinetic characterization of the peptidase activity of Escherichia coli Lon reveals the mechanistic similarities in ATP-dependent hydrolysis of peptide and protein substrates. Biochemistry, 41, 9418−9425.
- Hilliard J.J., Simon L.D., Van Melderen L., Maurizi M.R. (1998) PinA inhibits ATP hydrolysis and energy-dependent protein degradation by Lon protease. J. Biol. Chem., 273, 524−527.
- Tomoyasu Т., Yamanaka K" Murata K., Suzaki Т., Bouloc P., Kato A., Niki H., Hiraga S., Ogura T. (1993) Topology and subcellular localization of FtsH protein in Escherichia coli. J. Bacteriol., 175(5), 1352−1357.
- Akiyama Y., Yoshihisa Т., Ito K. (1995) FtsH, a membrane-bound ATPase, forms a complex in the cytoplasmic membrane of Escherichia coli. J. Biol. Chem., 270(40), 23 485−23 490.
- Herman C., Lecat S., D’Ari R, Bouloc P. (1995) Regulation of the heat-shock response depends on divalent metal ions in an hflB mutant of Escherichia coli. Mol. Microbiol., 18(2), 247−255.
- Saikawa N., Ito K., Akiyama Y. (2002) Identification glutamic acid 479 as the gluzincin coordinator of zinc in FtsH (HflB). Biochemistry, 41, 1861−1868.
- Shotland Y., Teff D., Koby S., Kobiler 0., Oppenheim, A.B. (2000) Characterization of a conserved alpha-helical, coiled-coil motif at the C-terminal domain of the ATP-dependent FtsH (HflB) protease of Escherichia coli. J. Mol. Biol, 299, 953−964.
- Akiyama Y" Kihara A., Tokuda H., Ito K. (1996) FtsH (HflB) is an ATP-dependent protease selectively acting on SecY and some other membrane proteins. J. Biol. Chem., 271(49), 31 196−31 201.
- Tomoyasu Т., Yamanaka K., Murata K., Suzaki Т., Bouloc P., Kato A., Niki H., Hiraga S., Ogura T. (1993) Topology and subcellular localization of FtsH protein in Escherichia coli. J. Bacteriol., 175(5), 1352−1357.
- Kihara A., Akiyama Y., Ito K. (1999) Dislocation of membrane proteins in FtsH-mediated proteolysis. EMBOJ. 18(11), 2970−2981.
- Akiyama, Y. and Ito, K. (2003) Reconstitution of membrane proteolysis by FtsH. J. Biol. Chem., 278,18 146−18 153.
- Chiba, S., Akiyama, Y. and Ito, K. (2002) Membrane protein degradation by FtsH can be initiated from either end. J. Bacteriol., 184,4775−4782.
- Kanemori M., Yanagi H., Yura T. (1999) Marked instability of the sigma(32) heat shock transcription factor at high temperature. Implications for heat shock regulation. J. Biol. Chem., 274(31), 22 002−22 007.
- Rist W., Jorgensen T.J., Roepstorff P., Bukau В., Mayer M.P. (2003) Mapping temperature-induced conformational changes in the Escherichia coli heat shock transcription factor sigma 32 by amide hydrogen exchange. J. Biol. Chem., 278(51), 51 415−51 421.
- Пб.Окипо Т., Yamada-Inagawa Т., Karata К., Yamanaka К., Ogura Т. (2004) Spectrometric analysis of degradation of a physiological substrate sigma32 by Escherichia coli AAA protease FtsH. J. Struct. Biol., 146(1−2), 148−54.
- Herman C., Thevenet D., D’Ari R., Bouloc, P. (1997) The HflB protease of Escherichia coli degrades its inhibitor lambda CHI. J., Bacteriol., 179, 358−363.
- Prajapati R.S., Ogura Т., Cutting S.M. (2000) Structural and functional studies on an FtsH inhibitor from Bacillus subtilis. Biochim. Biophys. Acta, 1475, 353−359.
- Hwang B.J., Woo K.M., Goldberg A.L., Chung C.H. (1988) Protease Ti, a new ATP-dependent protease in Escherichia coli, contains protein-activated ATPase and proteolytic functions in distinct subunits. J. Biol. Chem., 263(18), 8727−8734.
- Gottesman S., Clark W.P., de Crecy-Lagard V., Maurizi M.R. (1993) ClpX, an alternative subunit for the ATP-dependent Clp protease of Escherichia coli. Sequence and in vivo activities. J. Biol. Chem., 268(30), 22 618−22 626.
- Maurizi M.R., Clark W.P., Kim S.H., Gottesman S. (1990) Clp P represents a unique family of serine proteases. J. Biol. Chem., 265(21), 12 546−12 552.
- Wang J., Hartling J.A., Flanagan J.M. (1998) Crystal structure determination of Escherichia coli ClpP starting from an EM-derived mask. J. Struct. Biol., 124(2−3), 151−163.
- Thompson M.W., Maurizi M.R. (1994) Activity and specificity of Escherichia coli ClpAP protease in cleaving model peptide substrates. J. Biol. Chem., 269(27), 18 201 -18 208.
- Maurizi M.R., Thompson M.W., Singh S.K., Kim S.H. (1994) Endopeptidase Clp: ATP-dependent Clp protease from Escherichia coli. Methods Enzymol., 244, 314−331.
- Lupas A., Flanagan J.M., Tamura Т., Baumeister W. (1997) Self-compartmentalizing proteases. Trends Biochem. Sci., 22(10), 399−404.
- Beuron F., Maurizi M.R., Belnap D.M., Kocsis E., Booy F.P., Kessel M., Steven A.C.(1998) At sixes and sevens: characterization of the symmetry mismatch of the ClpAP chaperone-assisted protease. J. Struct. Biol., 123(3), 248−259.
- Levchenko I., Smith C.K., Walsh N.P., Sauer R.T., Baker T.A. (1997) PDZ-like domains mediate binding specificity in the Clp/HsplOO family of chaperones and protease regulatory subunits. Cell, 91, 939−947.
- Ortega J., Singh S.K., Ishikawa Т., Maurizi M.R. and Steven A.C. (2000) Visualization of substrate binding and translocation by the ATP-dependent protease, ClpXP. Mol. Cell, 6, 1515−1521.
- Lee C" Schwartz M.P., Prakash S., Iwakura M. and Matouschek A. (2001) ATP-dependent proteases degrade their substrates by processively unraveling them from the degradation signal. Mol. Cell, 7, 627−637.
- Reid B.G., Fenton W.A., Homwich A.L. and Weber-Ban E.U. (2001) ClpA mediates directional translocation of substrate proteins into the ClpP protease. Proc. Natl. Acad. Sci. USA (2001) 98, 3768−3772.
- Arribas J., Castano J.G. (1993) A comparative study of the chymotrypsin-like activity of the rat liver multicatalytic proteinase and the ClpP from Escherichia coli. J. Biol. Chem., 268, 21 165−21 171.
- Halperin Т., Ostersetzer O. and Adam Z. (2001) ATP-dependent association between subunits of Clp protease in pea chloroplasts. Planta, 213, 614−619.
- Kang M.S., Lim B.K., Seong I.S., Seol J.H., Tanahashi N., Tanaka K., Chung C.H. (2001) The ATP-dependent CodWX (HslVU) protease in Bacillus subtilis is an N-terminal serine protease. EMBO J., 20(4), 734−742.
- Yoo S.J., Shim Y.K., Seong I.S., Seol J.H., Kang M.S., Chung C.H. (1997) Mutagenesis of two N-terminal Thr and five Ser residues in HsIV, the proteolytic component of the ATP-dependent HslVU protease. FEBS Lett., 412(1), 57−60.
- Rohrwild M., Pfeifer G., Santarius U" Muller S.A., Huang H.C., Engel A., Baumeister W., Goldberg A.L. (1997) The ATP-dependent HslVU protease from Escherichia coli is a four-ring structure resembling the proteasome. Nat. Struct. Biol., 4(2), 133−139.
- Yoo S.J., Seol J.H., Seong I.S., Kang M.S., Chung C.H. (1997) ATP binding, but not its hydrolysis, is required for assembly and proteolytic activity of the HslVU protease in Escherichia coli. Biochem. Biophys. Res. Commun., 238(2), 581−585.
- Bochtler M., Hartmann C., Song H.K., Bourenkov G.P., Bartunik H.D., Huber R. (2000) The structures of HslU and the ATP-dependent protease HsIU-HsIV. Nature, 403(6771), 800−805.
- Bochtler M., Ditzel L., Groll M., Huber R. (1997) Crystal structure of heat shock locus V (HsIV) from Escherichia coli. Proc. Natl. Acad. Sci. USA, 94(12), 6070−6074.
- Lowe J., Stock D., Jap В., Zwickl P., Baumeister W., Huber R. (1995) Crystal structure of the 20S proteasome from the archaeon T. acidophilum at 3.4 A resolution. Science, 268(5210), 533−539.
- Groll M., Brandstetter H., Bartunik H., Bourenkow G., Huber R. (2003) Investigations on the maturation and regulation of archaebacterial proteasomes. J. Mol. Biol., 327(1), 75−83.
- Pickart C.M. (1997) Targeting of substrates to the 26S proteasome. FASEB J., 11(13), 10 551 066.
- Grziwa A., Baumeister W., Dahlmann В., Kopp F. (1991) Localization of subunits in proteasomes from Thermoplasma acidophilum by immunoelectron microscopy. FEBS Lett., 290(1−2), 186−190.
- Seemiiller E., Lupas A., Stock D., Lowe J., Huber R. and Baumeister W. (1995) Proteasome from Thermoplasma acidophilum: a threonine protease. Science, 268, 579−582.
- Groll M., Huber R. (2003) Substrate access and processing by the 20S proteasome core particle. Int. J. Biochem. Cell Biol., 35(5), 606−616.
- Maupin-Furlow J.A., Gil M.A., Karadzic I.M., Kirkland P.A., Reuter C.J. (2004) Proteasomes: perspectives from the Archaea. Front. Biosci., 9, 1743−1758.
- Kisselev A.F., Songyang Z., Goldberg A.L. (2000) Why does threonine, and not serine, function as the active site nucleophile in proteasomes? J. Biol. Chem., 275(20), 14 831−14 837.
- Zwickl P., Seemuller E., Kapelari В., Baumeister W. (2001) The proteasome: a supramolecular assembly designed for controlled proteolysis. Adv. Protein Chem., 59, 187 222.
- Claverol S., Burlet-Schiltz O., Girbal-Neuhauser E., Gairin J.E., Monsarrat B. (2002) Mapping and structural dissection of human 20 S proteasome using proteomic approaches. Mol. Cell. Proteomics, 1(8), 567−578.
- Heinemeyer W., Fischer M., Krimmer Т., Stachon U., Wolf D.H. (1997) The active sites of the eukaryotic 20 S proteasome and their involvement in subunit precursor processing. J. Biol. Chem., 272(40), 25 200−25 209.
- Arendt C.S., Hochstrasser M. (1997) Identification of the yeast 20S proteasome catalytic centers and subunit interactions required for active-site formation. Proc. Natl. Acad. Sci. USA, 94(14), 7156−7161.
- Kisselev A.F., Akopian T.N., Castillo V., Goldberg A.L. (1999) Proteasome active sites allosterically regulate each other, suggesting a cyclical bite-chew mechanism for protein breakdown. Mol. Cell, 4(3), 395−402.
- Zwickl P., Ng D" Woo K.M., Klenk H.P., Goldberg A.L. (1999) An archaebacterial ATPase, homologous to ATPases in the eukaryotic 26 S proteasome, activates protein breakdown by 20 S proteasomes. J. Biol. Chem., 274(37), 26 008−26 014.
- Dubiel W., Ferrell K., Pratt G., Rechsteiner M. (1992) Subunit 4 of the 26 S protease is a member of a novel eukaryotic ATPase family. J. Biol. Chem., 267(32), 22 699−22 702.
- WolIenberg K., Swaffield J.C. (2001) Evolution of proteasomal ATPases. Mol. Biol. Evol., 18(6), 962−974.
- Waxman L., Fagan J.M., Goldberg A.L. (1987) Demonstration of two distinct high molecular weight proteases in rabbit reticulocytes, one of which degrades ubiquitin conjugates. J. Biol. Chem., 262(6), 2451−2457.
- Hough R., Pratt G., Rechsteiner M. (1987) Purification of two high molecular weight proteases from rabbit reticulocyte lysate. J. Biol. Chem., 262(17), 8303−8313.
- Eytan E., Ganoth D., Armon Т., Hershko A. (1989) ATP-dependent incorporation of 20S protease into the 26S complex that degrades proteins conjugated to ubiquitin. Proc. Natl. Acad. Sci. USA, 86(20), 7751−7755.
- Hochstrasser M. (1996) Ubiquitin-dependent protein degradation. Annu. Rev. Genet., 30, 405−439.
- Haas A.L., Siepmann T.J. (1997) Pathways of ubiquitin conjugation. FASEB J., 11(14), 1257−1268.
- Gottesman S., Maurizi M.R., Wickner S. (1997) Regulatory subunits of energy-dependent proteases. Cell, 91(4), 435−438.
- Yamada-Inagawa Т., Okuno Т., Karata K., Yamanaka K., Ogura T. (2003) Conserved pore residues in the AAA protease FtsH are important for proteolysis and its coupling to ATP hydrolysis. J. Biol. Chem., 278(50), 50 182−7.
- Hershko A., Ciechanover A. (1998) The ubiquitin system. Ann. Rev. Biochem., 67,425−479.
- Lee D.H., Goldberg A.L. (1998) Proteasome inhibitors: valuable new tools for cell biologists. Trends Cell. Biol., 8(10), 397−403.
- Ciechanover A., Heller H., Katz-Etzion R., Hershko A. (1981) Activation of the heat-stable polypeptide of the ATP-dependent proteolytic system. Proc. Natl. Acad. Sci. USA, 78(2), 761 765.
- Hershko A., Ciechanover A., Rose I.A. (1981) Identification of the active amino acid residue of the polypeptide of ATP-dependent protein breakdown. J. Biol. Chem., 256(4), 1525−1528.
- Chau V., Tobias J.W., Bachmair A., Marriott D., Ecker D.J., Gonda D.K., Varshavsky A. (1989) Ubiquitin chain is confined to specific lysine in a targeted short-lived protein. Science, 243(4898), 1576−1583.
- Hershko A. (1996) Lessons from the discovery of the ubiquitin system. Trends Biochem. Sci., 21(11), 445−449.
- Hershko A., Ciechanover A. (1992) The ubiquitin system for protein degradation. Ann. Rev. Biochem., 61,761−807.
- Pickart C.M., Rose I.A. (1985) Functional heterogeneity of ubiquitin carrier proteins. J. Biol. Chem., 260(3), 1573−1581.
- Hadari Т., Warms J.V., Rose I.A., Hershko A. (1992) A ubiquitin C-terminal isopeptidase that acts on polyubiquitin chains. Role in protein degradation. J. Biol. Chem., 267(2), 719 727.
- Voges D., Zwickl P., Baumeister W. (1999) The 26S proteasome: a molecular machine designed for controlled proteolysis. Ann. Rev. Biochem., 68, 1015−1068.
- Kannan Y., Koga Y., Inoue Y., Haruki M., Takagi M., Imanaka Т., Morikawa M., Kanaya S. (2001) Active subtilisin-like protease from a hyperthermophilic archaeon in a form with a putative prosequence. Appl. Environ. Microbiol., 67(6), 2445−52.
- Du X., Choi I.G., Kim R., Wang W., Jancarik J., Yokota H., Kim SH. (2000) Crystal structure of an intracellular protease from Pyrococcus horikoshii at 2-A resolution. Proc. Natl. Acad. Sci. USA, 97(26), 14 079−84.
- Chang L.S., Hicks P.M., Kelly R.M. (2001) Protease I from Pyrococcus furiosus. Methods Enzymol., 330,403−13.
- Halio S.B., Bauer M.W., Mukund S., Adams M., Kelly R.M. (1997) Purification and Characterization of Two Functional Forms of Intracellular Protease Pfpl from the Hyperthermophilic Archaeon Pyrococcus furiosus. Appl. Environ. Microbiol., 63(1), 289−295.
- Громов Б.В. Удивительный мир архей. (1997) Соросовский образовательный журнал. 4, 23−26.
- De Castro R.E., Maupin-Furlow J.A., Gimenez M.I., Herrera Seitz M.K., Sanchez J.J. (2006) Haloarchaeal proteases and proteolytic systems. FEMS Microbiol. Rev., 30(1), 17−35.
- Rose R.W., Bruser T, Kissinger J.C., Pohlschroder M. (2002) Adaptation of protein secretion to extremely high-salt conditions by extensive use of the twin-arginine translocation pathway. Mol. Microbiol., 45(4), 943−50.
- Tamura N., Lottspeich F., Baumeister W., Tamura T. (1998) The role of tricorn protease and its aminopeptidase interacting factors in cellular protein degradation. Cell, 95(5), 637−48.
- Bosch J., Tamura Т., Bourenkov G., Baumeister W., Essen L.O. (2001) Purification, crystallization, and preliminary X-ray diffraction analysis of the Tricorn protease hexamer from Thermoplasma acidophilum. J. Struct. Biol., 134(1), 83−7.
- Groll M., Bochtler M., Brandstetter H., Clausen Т., Huber R. (2005) Molecular machines for protein degradation. Chembiochem., 6(2), 222−56.
- Yao T, Cohen R.E. (1999) Giant proteases: beyond the proteasome. Curr. Biol., 12- 9(15), 551−3.
- Chen L., Brugger K., Skovgaard M" Redder P., She Q, Torarinsson E., Greve B, Awayez M., Zibat A., Klenk H.P., Garrett R.A. (2005) The Genome of Sulfolobus acidocaldarius, a Model Organism of the Crenarchaeota. J. Bacteriol., 187(14), 4992−4999.
- Lin X., Fusek M., Tang J. (1991) Thermopsin, a thermostable acid protease from Sulfolobus acidocaldarius. Adv. Exp. Med. Biol., 306,255−7.
- Maupin-Furlow JA, Gil MA, Humbard MA, Kirkland PA, Li W, Reuter CJ, Wright AJ.2005) Archaeal proteasomes and other regulatory proteases. Curr. Opin. Microbiol., 8(6), 720−8.
- Maupin-Furlow JA, Gil MA, Karadzic IM, Kirkland PA, Reuter CJ. (2004) Proteasomes: perspectives from the Archaea. Front. Biosci., 9, 1743−58.
- Zwickl P., Ng D., Woo K.M., Klenk H.P., Goldberg A.L. (1999) An archaebacterial ATPase, homologous to ATPases in the eukaryotic 26 S proteasome, activates protein breakdown by 20 S proteasomes. J. Biol. Chem., 274(37), 26 008−26 014.
- Reuter CJ, Kaczowka SJ, Maupin-Furlow JA. (2004) Differential regulation of the Pan A and PanB proteasome-activating nucleotidase and 20S proteasomal proteins of the haloarchaeon Haloferax volcanii. J. Bacteriol., 186(22), 7763−7772.
- Zwickl P., Seemuller E., Kapelari В., Baumeister W. (2001) The proteasome: a supramolecular assembly designed for controlled proteolysis. Adv. Protein Chem., 59, 187 222.
- Kisselev A.F., Songyang Z., Goldberg A.L. (2000) Why does threonine, and not serine, function as the active site nucleophile in proteasomes? J. Biol. Chem., 275(20), 14 831−14 837.
- Maupin-Furlow JA, Humbard MA, Kirkland PA, Li W, Reuter С J, Wright AJ, Zhou G.2006) Proteasomes from structure to function: perspectives from Archaea. Curr. Top. Dev. Biol., 75,125−69.
- Метелина A. J1. (2001) Жгутики прокариот как система биологической подвижности. Успехи биол. химии, 41, 229−282.
- Albers S.V., Szabo Z., Driessen A.J. (2003) Archaeal homolog of bacterial type IV prepilin signal peptidases with broad substrate specificity. J. Bacteriol., 185(13), 3918−25
- Bardy SL, Jarrell KF. (2003) Cleavage of preflagellins by an aspartic acid signal peptidase is essential for flagellation in the archaeon Methanococcus voltae. Mol. Microbiol., 50(4), 133 947.
- Szabo Z., Albers S.V., and Driessen A.J. (2006) Driessen active-site residues in the type IV prepilin peptidase homologue PibD from the archaeon Sulfolobus solfataricus. J. Bacteriol., 188(4), 1437−43.
- Lemberg MK, Menendez J, Misik A, Garcia M, Koth CM, Freeman M. (2005) Mechanism of intramembrane proteolysis investigated with purified rhomboid proteases. EMBO J., 24(3), 464−72.
- Ротанова T.B. (2002) Пептидгидролазы с каталитической диадой Ser Lys. Сходство и различия активных центров АТР-зависимых Lon-протеиназ, репрессоров LexA, сигнальных пептидаз и протеиназ С-концевого процессинга. Вопр. мед. химии, 48(6), 541−552.
- Wagner I., van Dyck L., Savel’ev A.S., Neupert W., Langer T. (1997) Autocatalytic processing of the ATP-dependent PIM1 protease: crucial function of a pro-region for sorting to mitochondria. EMBO J., 16(24), 7317−7325.
- Chin D.T., GofF S.A., Webster Т., Smith Т., Goldberg A.L. (1988) Sequence of the lon gene in Escherichia coli. A heat-shock gene which encodes the ATP-dependent protease La. J. Biol. Chem., 263(24), 11 718−11 728.
- Ротанова Т.В. (2006) Протеолиз, сопряженный с гидролизом АТР. Структурно-функциональное исследование Ьоп-протеиназы Escherichia coli. Диссертация на соискание ученой степени доктора химических наук,
- Birghan С., Mundt Е., Gorbalenya А.Е. (2000) A non-canonical Lon proteinase lacking the ATPase domain employs the Ser-Lys catalytic dyad to exercise broad control over the life cycle of a double-stranded RNA virus. EMBOJ., 19(1), 114−123.
- Besche H., Tamura N., Tamura Т., Zwickl P. (2004) Mutational analysis of conserved AAA+ residues in the archaeal Lon protease from Thermoplasma acidophilum. FEBS Lett., 574(1−3), 161−166.
- Fukui Т., Eguchi Т., Atomi H., Imanaka T. (2002) A membrane-bound archaeal Lon protease displays ATP-independent proteolytic activity towards unfolded proteins and ATP-dependent activity for folded proteins. J. Bacteriol., 184(13), 3689−3698.
- Besche H., Zwickl P. (2004) The Thermoplasma acidophilum Lon protease has a Ser-Lys dyad active site. Eur. J. Biochem., 271(22), 4361−4365.
- Novy R., Drott D., Yaeger K., Mierendorf R. (2001) Overcoming the codon bias of E. coli for enhanced protein expression. inNovations, 12, 1−3.
- Nakamura Y., Gojobori Т., Ikemura T. (2000) Codon usage tabulated from international DNA sequence databases: status for the year. Nucl. Acids. Res., 28, 1.
- Goldberg A.L., Moerschell R.P., Chung C.H., Maurizi M.R. (1994) ATP-dependent protease La (lon) from Escherichia coli. Methods Enzymol., 244, 350−375.
- Rasulova F.S., Dergousova N.I., Starkova N.N., Melnikov E.E., Rumsh L.D., Ginodman L.M., Rotanova T.V. (1998) The isolated proteolytic domain of Escherichia coli ATP-dependent protease Lon exhibits the peptidase activity. FEBS Lett., 432(3), 179−181.
- Расулова Ф.С. (1998) Структурно-функциональное исследование АТР-зависимой Lon-протеиназы Escherichia coli. Диссертация на соискание ученой степени кандидата биологических наук.
- Мельников Э.Э., Цирульников К. Б., Ротанова Т. В. (2001) Сопряжение протеолиза и гидролиза АТР при функционировании Lon-протеиназы Escherichia coli. II. Гидролиз АТР и активность пептидгидролазных центров фермента. Биоорган, химия, 27(2), 124 133.
- Цирульников К.Б., Мельников Э. Э., Ротанова Т. В. (2003) Протеолиз, сопряженный с гидролизом АТР. Регуляция активности протеолитических центров Lon-протеиназы Escherichia coli. Биоорган, химия, 29(5), 486−494.
- Jones C.H., Bolken T. C, Jones K.F., Zeller G.O., Hruby D.E. (2001) Conserved DegP protease in gram-positive bacteria is essential for thermal and oxidative tolerance and full virulence in Streptococcus pyogenes. Infect Immun., 69(9), 5538−45.
- Bieniossek C., Schalch Т., Bumann M., Meister M., Meier R., Baumann U. (2006) The molecular architecture of the metalloprotease FtsH. Proc. Natl. Acad. Sci. USA, 103(9) — 306 671.
- Цирульников К.Б. (2001) Исследование взаимодействия протеолитического и АТР-азного центров нативной Lon-протеиназы Escherichia coli и ее мутантных форм. Диссертация на соискание ученой степени кандидата химических наук.
- Erzberger J.P., Berger J.M. (2006) Evolutionary relationships and structural mechanisms of AAA+ proteins. Annu. Rev. Biophys. Biomol. Struct., 35- 93−114.
- Rudyak S.G., Brenowitz M., Shrader Т.Е. (2001) Mg2±linked oligomerization modulates the catalytic activity of the Lon (La) protease from Mycobacterium smegmatis. Biochemistry, 40(31) — 9317−23.
- Lee AY, Tsay SS, Chen MY, Wu SH. (2004) Identification of a gene encoding Lon protease from Brevibacillus thermoruber WR-249 and biochemical characterization of its thermostable recombinant enzyme. Eur. J. Biochem., 271(4) — 834−44.
- Suzuki CK, Kutejova E, Suda K, (1995) Analysis and purification of ATP-dependent mitochondrial lon protease of Saccharomyces cerevisiae. Methods Enzymol., 260- 486−94.
- Goldberg A.L., Moerschell R.P., Chung C.H., Maurizi M.R. (1994) ATP-dependent protease La (lon) from Escherichia coli. Methods Enzymol., 244, 350−375.
- Leffers G., Rasulova F., Kessel M., Steven A.C., Leapman R., Gottesman S., Maurizi M.R. (1999) Organization of functional and structural domains in E. coli Lon protease. Meeting on Biology of Proteolysis. Abstracts. Cold Spring Harbor, New York, 52.
- Lee AY, Hsu CH, Wu SH. (2004) Functional domains of Brevibacillus thermoruber lon protease for oligomerization and DNA binding: role of N-terminal and sensor and substrate discrimination domains. J. Biol. Chem., 279(33), 34 903−12.
- Bradford M.M. (1976) A rapid and sensitive method for the quantitation of microgram quantities of protein utilizing the principle of protein-dye binding. Anal. Biochem., 72, 248 254.
- Laemmli U.K. (1970) Cleavage of structural proteins during the assembly of the head of bacteriophage T4. Nature, 227(5259) — 680−685.
- Bencini D.A., Wild J.R., O’Donovan G.A. (1983) Linear one-step assay for the determination of ortophospate. Anal Biochem., 132(2) — 254−258.
- Lanzetta P.A., Alvarez L.J., Reinach P. S. and Candia O.A. (1979) An improved assay for nanomole amounts of inorganic phosphate. Anal. Biochem., 100(1) — 95−7.