Изучение генетического контроля RecA-независимой индукции профага ?
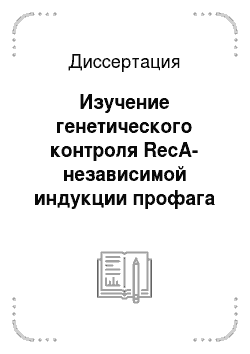
Диссертация
Предполагается, что индукция профага, опосредованная SOS системой, служит цели спасения фага, когда клетке грозит гибель при возникновении повреждений в ДНК. В настоящее время известны воздействия окружающей среды, такие как осмотический шок, изменение кислотности среды и т. д., которые не действуют на ДНК, но являются также губительными для клетки. Можно допустить, что такие стрессы способны… Читать ещё >
Содержание
- I. ОБЗОР ЛИТЕРАТУРЫ: «Адаптация клеток Escherichia coli к воздействиям окружающей среды»
- 1. Введение
- 2. Схемы сигнальной трансдукции в бактериях
- 2. 1. Внутриклеточная передача сигналов через коммуникативные модули
- 2. 2. Фосфорилирующие активности сенсоров и эффекторов
- 2. 3. Сигнальные свойства сенсоров и эффекторов
- 2. 4. Осморегуляция
- 2. 5. Ассимиляция азота
- 2. 6. Хемотаксис
- 3. Регуляция синтеза капсульного полисахарида в. клетках
- Escherichia coli К
- 3. 1. Введение
- 3. 2. Регуляция: обзор
- 3. 3. RcsB и RcsC составляют двухкомпонентную регуляторную систему
- 3. 4. Lon расщепляет RcsA
- 3. 5. RcsA взаимодействует с RcsB для стимуляции транскрипции генов cps
- 3. 6. Модель синтеза клеточной капсулы
- 4. SOS ответ клеток Escherichia col
- 4. 1. Введение
- 4. 2. SOS система
- 4. 3. Развитие модели регуляции SOS системы
- 4. 4. Тонкая настройка SOS системы
- 5. Выживание клеток при голодании и стрессах в стационарной фазе развития
- 5. 1. Введение
- 5. 2. Ген rpoS кодирует регулятор стационарной фазы, имеющий сходство с вегетативными, а факторами
- 5. 3. RpoS-зависимые гены и их клеточные функции
- 5. 4. Как RpoS контролирует генную экспрессию
- 5. 5. Перспективы
- II. МАТЕРИАЛЫ И МЕТОДЫ
- 1. Среды и химические вещества
- 2. Бактериальные штаммы и бактериофаги
- 3. Плазмиды
- 4. Перенос мутации из плазмиды в хромосому
- 5. Спот-тест и количественное определение эффективности индукции профагаА
- 6. Определение эффективности адсорбции фага к
- III. РЕЗУЛЬТАТЫ
- 1. Выделение неиндуцибельных (1псГ) мутантов, генетическое картирование мутаций и комплементационный анализ
- 2. Клонирование генов Escherichia coli, чья сверхэкспрессия приводит к
- 11. есА-независимой индукции профага X
- 2. 1. Избыток ЯсэА и БбгА в клетке приводит к ЯесА-независимой индукции профага X
- 2. 2. Эффект сверхпродукции ЯсэА и ЭбгА на индукцию других лямбдоидных профагов
- 2. 3. Количественное определение эффективности ЯесА-независимой индукции профага X
- 2. 4. Роль генов г а-А, гс$В и (кгА в ЯесА-независимой индукции профага X
- 2. 5. Эффект сверхпродукции репрессора фага X на индукцию лямбдоидных профагов посредством системы ЯсзАВС
- 2. 6. Механизм индукции профага X посредством ЯсбА и БбгА РНК
- IV. ОБСУЖДЕНИЕ РЕЗУЛЬТАТОВ
- ВЫВОДЫ
Список литературы
- Aiba, Н., Т. Mizuno, and S. Mizushima. 1989a. Transfer of phosphoryl group between two regulatory proteins involved in osmoregulatory expression of the ompF and ompC genes in Escherichia coli. J. Biol. Chem. 264:8563−8567.
- Aiba, H., F. Nakasai, S. Mizushima, and T. Mizuno. 19 896. Evidence for the physiological importance of the phosphotransfer between the two regulatory components, EnvZ and OmpR, in osmoregulation in Escherichia coli. J. Biol. Chem. 264:14 090−14 094.
- Aiba, H., F. Nakasai, S. Mizushima, and T. Mizuno. 1989c. Phosphorylation of a bacterial activator protein, OmpR, by a protein kinase, EnvZ, results in stimulation of its DNA-binding ability. J. Biochem. 106:5−7.
- Aldea, M., T. Garrido, C. Hernandez-Chico, M. Vicente, and S. R. Kushner. 1989. Induction of a growth-phase-dependent promoter triggers transcription of bolA, an Escherichia coli morphogene. EMBO J. 8:3923−3931.
- Allen, P., C. A. Hart, and J. R. Saunders. 1987. Isolation «from Klebsiella and characterization of two res genes that activate colanic acid capsular biosynthesis in Escherichia coli. J. Gen. Microbiol. 133:331−340.
- Almiron, M., A. Link, D. Furlong, and R. Kolter. 1992. A novel DNA-binding protein with regulatory and protective roles in starved Escherichia coli. Genes Dev. 6:2646−2654.
- Arnosti, D. N., and M. J. Chamberlin. 1989. Secondary a factor controls transcription of flagellar and chemotaxis genes in Escherichia coli. Proc. Natl. Acad. Sci. USA 86:830 834.
- Atlund, Т., A. Nielsen, and F. G. Hansen. 1989. Isolation, characterization, and nucleotide sequence of app Y, a regulatory gene for growth-phase-dependent gene expression in Escherichia coli. J. Bacteriol. 171:1683−1691.
- Bagg, A., C. J. Kenyon, and G. C. Walker. 1981. Inducibility of a gene product required for UV and chemical mutagenesis in Escherichia coli. Proc. Natl. Acad. Sci. USA 78:5749−5753.
- Barak, R., and M. Eisenbach. 1992. Correlation between phosphorylation of the chemotaxis protein CheY and its activity at the flagellar motor. Biochemistry 31:18 211 826.
- Berg, H. C. 1988. A physicist looks at bacterial chemotaxis. Cold Spring Harbor Symp. Quant. Biol. 53:1−9.
- Boos, W., U. Ehmann, E. Bremer, A. Middendorf, and P. Postma. 1987. Trehalose of Escherichia coli. J. Biol. Chem. 262:13 212−13 218.
- Borkovich, K. A., N. Kaplan, J. F. Hess, and M. I. Simon. 1989. Transmembrane signal transduction in bacterial chemotaxis involves ligand-dependent activation of phosphate group transfer. Proc. Natl. Acad. Sci. USA 86:1208−1212.
- Bourret, R. B., K. A. Borkovich, and M. I. Simon. 1991. Signal transduction pathways involving protein phosphorylation in prokaryotes. Annu. Rev. Biochem. 60:401−441.
- Bourret, R. B., J. Davagnino, and M. I. Simon. 1993. The carboxy-terminal portion of the CheA kinase mediates regulation of autophosphorylation by transducer and CheW. J. Bacteriol. 175:2097−2101.
- Bourret, R. B., J. F. Hess, and M. I. Simon. 1990. Conserved aspartate residues and phosphorylation in signal transduction by the chemotaxis protein CheY. Proc. Natl. Acad. Sci. USA 87:41−45.
- Brent, R. 1982. Regulation and autoregulation by lexA protein. Biochimie 64:565−569.
- Brent, R., and M. Ptashne. 1980. The I ex A gene product represses its own promoter. Proc. Natl. Acad. Sci. USA 77:1932−1936.
- Brent, R., and M. Ptashne. 1981. Mechanism of action of the lexA gene product. Proc. Natl. Acad. Sci. USA 78:4202−4208.
- Brill, J. A., C. Quinlan-Walshe, and S. Gottesman. 1988. Fine-structure mapping and identification of two regulators of capsule synthesis in Escherichia coli K-12. J. Bacteriol. 170:2599−2611.
- Brissette, R. E., K. 1. Tsung, and M. Inouye. 1991. Supression of a mutation in OmpR at the putative phosphorylation center by a mutant EnvZ protein in Escherichia coli. J. Bacteriol. 173:601−608.
- Campbell, A. 1961. Conditions for existence of bacteriophage. Evolution 15:153−165.
- Casaregola, S., R. D’Ari, and O. Huisman. 1982. Quantitative evaluation of recA gene expression in Escherichia coli. Mol. Gen Genet. 185:430−439.
- Close, T. J., and R. L. Rodriguez. 1982. Construction and characterization of the chloramphenicol-resistance gene cartridge: a new approach to the transcriptional mapping of extrachromosomal elements. Gene 20:305−316.
- Csonka, L. N. 1989. Physiological and genetic responses of bacteria to osmotic stress. Microbiol. Rev. 53:121−147.
- Daniels, D. L., F. Sanger, and A. R. Coulson. 1983. Features of bacteriophage X: analysis of the complete nucleotide sequence. Cold Spring Harbor Symp. Quant. Biol. 48:1009−1024.
- Defais, M., P. Fauquet, M. Radman, and M. Errera. 1971. Ultraviolet reactivation and ultraviolet mutagenesis of lambda in different genetic systems. Virology 43:495−503.
- Demple, B., and C. F. Amabile-Cuevas. 1991. Redox redux: The control of oxidative stress responses. Cell 67:837−839.
- Dersch, P., K. Schmidt, and E. Bremer. 1993. Synthesis of the Escherichia coli K-12 nucleoid-associated DNA-binding protein H-NS is subject to growth-phase control and autoregulation. Mol. Microbiol. 8:875−889.
- Diaz-Guerra, L., F. Moreno, and J. L. SanMillan. 1989. appR gene product activates transcription of microcin C7 plasmid genes. J. Bacteriol. 171:2906−2908.
- Eisenbach, M. 1991. Signal transduction in bacterial chemotaxis, p. 131−202. In J. L. Spudich (ed.), Sensory receptors and signal transduction. Wiley-Liss, Inc., New York.
- Elespuru, R. K. 1984. Induction of bacteriophage lambda by DNA-interacting chemicals, p. 213−231. In F. J. de Serres (ed.), Chemical Mutagens, vol. 9. Plenum Press, New York.
- Farr, S. B., and T. Kogoma. 1991. Oxidative stress responses in Escherichia coli and Salmonella typhimurium. Microbiol. Rev. 55:561−585.
- Feng, J., M. R. Atkinson, W. McCleary, J. B. Stock, B. L. Wanner, and A. J. Ninfa. 1992. Role of phosphorylated metabolic intermediates in the regulation of glutamine synthetase synthesis in Escherichia coli. J. Bacteriol. 174:6061−6070.
- Forst, S., J. Delgado, and M. Inouye. 1989. Phosphorylation of OmpR by the osmosensor EnvZ modulates expression of the ompF and ompC genes in Escherichia coli. Proc. Natl. Acad. Sci. USA 86:6052−6056.
- Forst, S., J. Delgado, A. Rampersaud, and M. Inouye. 1990. In vivo phosphorylation of OmpR, the transcription activator of the ompF and ompC genes in Escherichia coli. J. Bacteriol. 172:3473−3477.
- Foster, P. L. 1992. Directed mutations: between unicorns and goats. J. Bacteriol. 174:1711−1716.
- Gegner, J. A., D. R. Graham, A. F. Roth, and F. W. Dahlquist. 1992. Assembly of an MCP receptor, CheW, and kinase CheA complex in the bacterial chemotaxis signal transduction pathway. Cell 70:975−982.
- Gervais, F. G., and G. R.Drapeau. 1992. Identification, cloning, and characterization of rcsF, a new regulator gene for exopolysaccharide synthesis that supresses the division mutation ftsZ84 in Escherichia coli K-12. J. Bacteriol. 174:8016−8022.
- Giaever, H. M., O. B. Styrvold, I. Kaasen, and A. R. Strom. 1988. Biochemical and genetic characterization of osmoregulatory trehalose synthesis in Escherichia coli. J. Bacteriol. 170:2841−2849.
- Gimble, F. S., and R. T. Sauer. 1985. Mutations in bacteriophage a, repressor that prevent RecA-mediated cleavage. J. Bacteriol. 162:147−154.
- Gimble, F. S., and R. T. Sauer. 1986. A repressor inactivation: properties of purified ind~ proteins in the autodigestion and RecA-mediated cleavage reactions. J. Mol. Biol. 192:39−47.
- Gottesman, S., M. Gottesman, J. E. Shaw, and M. L. Pearson. 1981. Protein degradation in E. coli: the lort mutation and bacteriophage lambda N and ell protein stability. Cell 24:225−233.
- Gottesman, S., and V. Stout. 1991. Regulation of capsular polysaccharide synthesis in Escherichia coli K12. Mol. Microbiol. 5:1599−1606.
- Gottesman, S., P. Trisler, and A. Torres-Cabassa. 1985. Regulation of capsular polysaccharide synthesis in Escherichia coli K-12: characterization of three regulatory genes. J. Bacteriol. 162:1111−1119.
- Groat, R. G., J. E. Schultz, E. Zychlinski, A. T. Bockman, and A. Matin. 1986. Starvation proteins in Escherichia coli: kinetics of synthesis and role in starvation survival. J. Bacteriol. 168:486−493.
- Groisman, E. A., and M. J. Casadaban. 1986. Mini-Mu bacteriophage with plasmid replicon for in vivo cloning and lac gene fusing. J. Bacteriol. 168:357−364.
- Hazelbauer, G. L. 1992. Bacterial chemoreceptors. Curr. Opin. Struct. Biol. 2:505−510.
- Hazelbauer, G. L., H. C. Berg, and P. Matsumura. 1993. Bacterial motility and signal transduction. Cell 73:15−22.
- Hazelbauer, G. L., R. Yaghmai, G. G. Burrows, J. W. Baumgartner, D. P. Dutton, and D. G. Morgan. 1990. Transducers: transmembrane receptor proteins involved in bacterial chemotaxis. Soc. Gen. Microbiol. Symp. 46:107−134.
- Hengge-Aronis, R. 1993a. The role of rpoS in early stationary phase gene regulation in Escherichia coli K12, p. 171−200. In S. Kjelleberg (ed.), Starvation in bacteria. Plenum Press, New York.
- Hengge-Aronis, R. 19 936. Survival of hunger and stress: the role of rpoS in early stationary phase regulation in E. coli. Cell 72:165−168.
- Hengge-Aronis, R., and D. Fischer. 1992. Identification and molecular analysis of glgS, a novel growth phase-regulated and rpoS-dependent gene involved in glycogen synthesis in Escherichia coli. Mol. Microbiol. 6:1877−1886.
- Hess, J. F., R. B. Bourret, and M. I. Simon. 1988a. Histidine phosphorylation and phoshporyl group transfer in bacterial chemotaxis. Nature 336:139−143.
- Hess, J. F., K. Oosawa, N. Kaplan, and M. I. Simon. 19 886. Phosphorylation of three proteins in the signaling pathway of bacterial chemotaxis. Cell 53:79−87.
- Higgins, C. F., J. C. D. Hinton, C. S. J. Hinton, T. Owen-Hughes, G. D. Pavitt, and A. Seirafi. 1990. Protein HI: a role for chromatin structure in the regulation of bacterial gene expression and virulence? Mol. Microbiol. 4:2007−2012.
- Horii, T., T. Ogawa, T. Nakatani, T. Hase, H. Matsubara, and H. Ogawa. 1981a. Regulation of SOS function: purification of E. coli LexA protein and determination of its specific site cleaved by the RecA protein. Cell 27:515−522.
- Horii, T., T. Ogawa, and H. Ogava. 19 816. Nucleotide sequence of the lexA gene of E. coli. Cell 23:689−697.
- Huisman, O., and R. D’Ari. 1981. An inducible DNA replication-cell division coupling mechanism in E. coli. Nature (London) 290:797−799.
- Igo, M. M., A. J. Ninfa, J. B. Stock, and T. J. Silhavy. 1989. Phosphorylation and dephosphorylation of bacterial transcriptional activator by a transmembrane receptor-Genes Dev. 3:1725−1734.
- Igo, M. M., J. M. Slauch, and T. J. Silhavy. 1990. Signal transduction in bacteria: kinases that control gene expression. New Biol. 2:5−9.
- Jann, K., and B. Jann. 1987. Polysaccharide antigens of Escherichia coli. Rev. Inf. E>lS-9 (suppl. 5):S517-S526.
- Jenkins, D. E., S. A. Chaisson, and A. Matin. 1990. Starvation-induced cross protection against osmotic challenge in Escherichia coli. J. Bacteriol. 172:2779−2781.
- Jenkins, D. E., J. E. Shultz, and A. Matin. 1988. Starvation-induced cross-protection against heat or H202 challenge in Escherichia coli. J. Bacteriol. 170:3910−3914.
- Jones, C. J., and S. Aizawa. 1991. The bacterial flagellum and flagellar motor: structure, assembly and function. Adv. Microbiol. Physiol. 32:109−172.
- Jung, J. U., C. Gutierrez, F. Martin, M. Ardourel, and M. Villarejo. 1990. Transcription of osmB, a gene encoding an Escherichia coli lipoprotein, is regulated by dual signals. J. Biol. Chem. 265:10 574−10 581.
- Keener, J., and S. Kustu. 1988. Protein kinase and phosphoprotein phosphotase activities of nitrogen regulatory proteins NTRB and NTRC of enteric bacteria: roles of conserved amino-terminal domain ofNTRC. Proc. Natl. Acad. Sci. USA 85:4976−4980
- Kenyon, C., and G. Walker. 1980. DNA-damaging agents stimulate gene expression at specific loci in Escherichia coli. Proc. Natl. Acad. Sci. USA 77:2819−2823.
- Kenyon, C., and G. Walker. 1981. Expression of the E. coli uvrA gene is inducible. Nature (London) 289:808−810.
- Kim, B., and J. W. Little. 1993. LexA and X cl repressors as enzymes: specific cleavage in an intermolecular reaction. Cell 73:1165−1173.
- Kohara, Y., K. Akiyama, and K. Isono. 1987. The physical map of the whole E. coli chromosome: application of a new strategy for rapid analysis and sorting of a large genomic library. Cell 50:495−508.
- Kolter, R., and F. Moreno. 1992. Genetics of ribosomally synthesized peptide antibiotics. Annu. Rev. Microbiol. 46:141−163.
- Krueger, J. H., S. J. Elledge, and G. C. Walker. 1983. Isolation and characterization of TnJ insertion mutations in the lexA gene of Escherichia coli. J. Bacteriol. 153:1368−1378.
- Lamont, I., A. M. Brumby, and J. B. Egan. 1989. UV induction of coliphage 186: prophage induction as an SOS function. Proc. Natl. Acad. Sci. USA 86:5492−5496.
- Lange, R., and R. Hengge-Aronis. 1991a. Growth phase-regulated expression of bolA and morphology of stationary-phase Escherichia coli cells are controlled by the novel sigma factor as (rpoS). J. Bacteriol. 173:4474−4481.
- Lange, R., and R. Hengge-Aronis. 19 916. Identification of a central regulator of stationary-phase gene expression in Escherichia coli. Mol. Microbiol. 5:49−59.
- Lin, L. L., and J. W. Little. 1988. Isolation and characterization of noncleavable (Ind~) mutants of the LexA repressor of Escherichia coli K-12. J. Bacteriol. 170:2163−2173.
- Lin, L. L., and J. W. Little. 1989. Autodigestion and RecA-dependent cleavage of Ind~ mutant LexA proteins. J. Mol. Biol. 210:439−452.
- Little, J. W. 1983. The SOS regulatory system: control of its state by the level of recA protease. J. Mol. Biol. 167:791−808.
- Little, J. W. 1984. Autodigestion of lexA and phage lambda repressors. Proc. Natl. Acad. Sei. USA 81:1375−1379.
- Little, J. W. 1991. Mechanism of specific LexA cleavage: autodigestion and the role of RecA coprotease. Biochimie 73:411−422.
- Little, J. W., and J. E. Harper. 1979. Identification of the I ex A gene product of Escherichia coli. Proc. Natl. Acad. Sei. USA 76:6147−6151.
- Little, J. W., D. W. Mount, and C. R. Yanisch-Perron. 1981. Purified lexA protein is a repressor of the recA and lexA genes. Proc. Natl. Acad. Sei. USA 78:4199−4203.
- Lloyd, R. G., S. M. Picksley, and C. Prescott. 1983. Inducible expression of a gene specific to the recF pathway for recombination in Escherichia coli Kl2. Mol. Gen. Genet. 190:162−167.
- Loewen, P. C., J. Switala, and B. L. Triggs-Raine. 1985. Catalases HPI and HPII in Escherichia coli are induced independently. Arch. Biochem. Biophys. 243:144−149.
- Loewen, P. C., and B. L. Triggs. 1984. Genetic mapping of katF, a locus that with katE affects the synthesis of a second catalase species in Escherichia coli. J. Bacteriol. 160:668 675.
- Lonetto, M., M. Gribskov, and C. A. Gross. 1992. The a70 family: sequence conservation and evolutionary relationships. J. Bacteriol. 174:3843−3849.
- Lukat, G. S., B. H. Lee, J. M. Mottonen, A. M. Stock, and J. B. Stock. 1991. Roles of the highly conserved aspartate and lysine residues in the response regulator of bacterial Chemotaxis. J. Biol. Chem. 266:8348−8354.
- Lukat, G. S., W. R. McCleary, A. M. Stock, and J. B. Stock. 1992. Phosphorylation of bacterial response regulator proteins by low molecular weight phospho-donors. Proc. Natl. Acad. Sci. USA 89:718−722.
- Lupas, A., and J. Stock. 1989. Phosphorylation of an N-terminal regulatory domain activates the CheB methylesterase in bacterial chemotaxis. J. Biol. Chem. 264:1 733 717 342.
- Lwoff, A. 1953. Lysogeny. Bacterid. Rev. 17:269−237.
- Lwoff, A., L. Siminovitch, and N. Kjeldgaard. 1950. Induction de la production de bacteriophages chez une bacterie lysogene. Ann. Inst. Pasteure 79:815−859 (In French).
- Macnab, R. B. 1992. Genetics and biogenesis of bacterial flagella. Annu. Rev. Genet. 26:131−158.
- Maddock, J. R., and L. Shapiro. 1993. Polar location of the chemoreceptor complex in the Escherichia coli. Science 259:1717−1723.
- Maeda, S., and T. Mizuno. 1990. Evidence for multiple OmpR-binding sites in the upstream activation sequence of the ompC promoter in Escherichia coli: a single OmpR-binding site is capable of activating the promoter. J. Bacteriol. 172:501−503.
- Manson, M. D. 1992. Bacterial motility and chemotaxis. Adv. Microbiol. Physiol. 33:277−346.
- Markovitz, A. 1964. Regulatory mechanisms for synthesis of capsular polysaccharide in mucoid mutants of Escherichia coli K12. Proc. Natl. Acad. Sci. USA 51:239−246.
- Matin, A. 1991. The molecular basis of carbon-starvation-induced general resistance in Escherichia coli. Mol. Microbiol. 5:3−10.
- McCallum, K. L., and C. Whitfield. 1991. The rcsA gene of Klebsiella pneumoniae 01: K20 is involved in expression of the serotype-specific K (capsular) antigen. Infecti. Immun. 59:494−502.
- McCann, M. P., J. P. Kidwell, and A. Matin. 1991. The putative a factor KatF has a central role in development of starvation-mediated general resistance in Escherichia coli. J. Bacteriol. 173:4188−4194.
- McEntee, K., and W. Epstein. 1977. Isolation and characterization of specialized transduicing bacteriophages for the recA gene of Escherichia coli. Virology 77:306−318.
- Merrick, M. J. 1993. In a class of its own-the RNA polymerase sigma factor a54 (aN). Mol. Microbiol. 10:903−909.
- Miller, J. H. 1972. Experiments in molecular genetics. Cold Spring Harbor Laboratory Press, Cold Spring Harbor, New York.
- Miller, H. I., M. Kirk, and H. Echols. 1981. SOS induction and autoregulation of the himA gene for site-specific recombination in E. coli. Proc. Natl. Acad. Sci. USA 78:67 546 758.
- Mizuno, T., and S. Mizushima. 1990. Signal transduction and gene regulation through the phosphorylation of two regulatory components: the molecular basis for the osmotic regulation of the porin genes. Mol. Microbiol. 4:1077−1082.
- Moreau, P., A. Bailone, and R. Devoret. 1976. Prophage X induction in Escherichia coli K12 envA uvrB: a highly sensitive test for potential carcinogens. Proc. Natl. Acad. Sci. USA 73:3700−3704.
- Mulvey, M. R., and P. C. Loewen. 1989. Nucleotide sequence of katF of Escherichia coli suggests KatF protein is a novel a transcription factor. Nucleic Acids Res. 17:99 799 991.
- Ninfa, A. J., and R. L. Bennett. 1991. Identification of the site of autophosphorylation of the bacterial protein kinase/phosphatase NRh. J. Biol. Chem. 266:6888−6893.
- Ninfa, A. J., and B. Magasanik. 1986. Covalent modification of the glnG product, NRi, by the glnL product, NRh, regulates the transcription of the glnALG operon in Escherichia coli. Proc. Natl. Acad. Sci. USA 83:5909−5913.
- Ninfa, A. J., L. J. Reitzer, and B. Magasanik. 1987. Initiation of transcription at the bacterial glnAp2 promoter by purified E. coli components is facilitated by enhancers. Cell 50:1039−1046.
- Ninfa, A. J., A. Stock, S. Mowbray, and J. Stock. 1991. Reconstitution of the bacterial chemotaxis signal transduction system from purified components. J. Biol. Chem. 266:9764−9770.
- Ogava, T., and J. I. Tomizava. 1967. Abortive lysogenization of bacteriophage lambda b2 and residual immunity of non-lysogenic segregants. J. Mol. Biol. 23:225−245.
- Olsen, A., A. Arnqvist, S. Sukupolvi, and S. Normark. 1993. The RpoS sigma factor relieves H-NS-mediated transcriptional repression of csgA, the subunit gene of fibronectin-binding curli m Escherichia coli. Mol. Microbiol. 7:523−536.
- Olsen, A., A. Jonsson, and S. Normark. 1989. Fibronectin binding mediated by a novel class of surface organells of Escherichia coli. Nature (London) 338:652−655.
- Oosawa, K., J. F. Hess, and M. I. Simon. 1988. Mutations defective in bacterial chemotaxis show modified protein phosphorylation. Cell 53:89−96.
- Ozaki, M., A. Wada, N. Fujita, and A. Ishihama. 1991. Growth phase-dependent modification of RNA polymerase in Escherichia coli. Mol. Gen. Genet. 230:17−23.
- Parker, B., and M. G. Marinus. 1988. A simple and rapid method to obtain substitution mutations in Escherichia colv. isolation of a dam deletion/insertion mutation. Gene 73:531 535.
- Parkinson, J. S. 1993. Signal transduction schemes of bacteria. Cell 73:857−871.
- Parkinson, J. S., and E. C. Kofoid. 1992. Communication modules in bacterial signaling proteins. Annu. Rev. Genet. 26:71−112.
- Phizicky, E. M., and J. W. Roberts. 1981. Induction of SOS functions: regulation of proteolytic activity of E. coli RecA protein by interaction with DNA and nucleoside triphosphate. Cell 25:259−267.
- Popham, D. L., D. Szeto, J. Keener, and S. Kustu. 1989. Function of a bacterial activator protein that binds to transcriptional enhancers. Science 243:629−635.
- Preiss, J. 1989. Chemistry and metabolism of intracellular reserves, p. 189−258. In J. C. Poindexter and E. R. Leadbetter (ed.), Bacteria in Nature. Plenum Press, New York.
- Preiss, J., and T. Romeo. 1989. Phisiology, biochemistry and genetics of bacterial glycogen synthesis. Adv. Microbiol. Phys. 30:183−238.
- Quiliardet, P., O. Huisman, R. D’Ari, and M. Hofnung. 1982. SOS chromotest, a direct assay of induction of an SOS function in Escherichia coli K-12 to measure genotoxicity. Proc. Natl. Acad. Sci. USA 79:5971−5975.
- Radding, C. M. 1982. Homologous pairing and strand exchange in genetic recombination. Annu. Rev. Genet. 16:405−437.
- Radman, M. 1975. SOS repair hypothesis: phenomenology of an inducible DNA repair which is accompanied by mutagenesis, p. 355−367. In P. Hanawalt and R. B. Setlow (ed.), Molecular mechanisms for repair of DNA, part A. Plenum Publishing Corp., New York.
- Raina, S., D. Missakas, and C. Georgopoulos. 1995. The rpoE gene encoding the sigma E (sigma 24) heat shock sigma factor of Escherichia coli. EMBO J. 14:1043−1055.
- Rampersaud, A., and M. Inouye. 1991. Procaine, a local anesthetic, signals through the EnvZ receptor to change the DNA binding affinity of the transcriptional activator protein OmpR. J. Bacteriol. 173:6882−6888.
- Rampersaud, A., S. Norioka, and M. Inouye. 1989. Characterization of OmpR binding sequences in the upstream region of the ompF promoter essential for transcriptional activation. J. Biol. Chem. 264:18 693−18 700.
- Retallack, D. M., and D. I. Friedman. 1995. A role for a small stable RNA in modulating the activity of DNA-binding proteins. Cell 83:227−235.
- Roberts, J., and R. Devoret. 1983. Lysogenic induction, p. 123−144. In R. W. Hendrix, J. W. Roberts, F. W. Stahl and R. A. Weisberg (ed.), Lambda II. Cold Spring Harbor Laboratory Press, Cold Spring Harbor, New York.
- Roland, K. L., and J. W. Little. 1990. Reaction of LexA repressor with diisopropyl fluorophosphate. A test of the serine protease model. J. Biol. Chem. 265:12 828−12 835.
- Roland, K. L., M. H. Smith, J. A. Rupley, and J. W. Little. 1992. In vitro analysis of mutant LexA proteins with an increased rate of specific cleavage. J. Mol. Biol. 228:395 408.
- Roman, S. J., M. Meyers, K. Volz, and P. Matsumura. 1992. A chemotactic signaling surface on CheY defined by suppressors of flagellar switch mutations. J. Bacteriol. 174:6247−6255.
- Ronson, C. W., B. T. Nixon, and F. M. Ausubel. 1987. Conserved domains in bacterial regulatory proteins that respond to environmental stimuli. Cell 49:579−581.
- Russo, F. D., and T. J. Silhavy. 1991. EnvZ controls the concentration of phosphorylated OmpR to mediate osmoregulation of the porin genes. J. Mol. Biol. 222:567−580.
- Sak, B. D., A. Eisenstark, and D. Touati. 1989. Exonuclease III and the catalase hydroperoxidase II in Escherichia coli are both regulated by the katF product. Proc. Natl. Acad. Sci. USA 86:3271−3275.
- Salles, B., and M. Defais. 1984. Signal of induction of RecA protein in Escherichia coli. Mutat. Res. 131:53−59.
- Sambrook, J., E. F. Fritsch, and T. Maniatis. 1989. Molecular cloning: a laboratory manual- 2-nd ed. Cold Spring Harbor Laboratory Press, Cold Spring Harbor, New York.
- Sanders, D. A., C. B. Gillece, A. L. Burlingame, and D. E., Jr. Koshland. 1992. Phosphorylation site of NtrC, a protein phosphatase whose covalent intermediate activates transcription. J. Bacteriol. 174:5117−5122.
- Sanders, D. A., B. L. Gillece-Castro, A. M. Stock, A. L. Burlingame, and D. E., Jr. Koshland. 1989. Identification of the site of phosphorylation of the chemotaxis response regulator protein, CheY. J. Biol. Chem. 264:21 770−21 778.
- Sassanfar, M., and J. W. Roberts. 1990. Nature of the SOS-inducing signal in Escherichia coli. The involvement of DNA replication. J. Mol. Biol. 212:79−96.
- Sasse-Dwight, S., and J. D. Gralla. 1988. Probing the Escherichia coli glnALG upstream activation mechanism in vivo. Proc. Natl. Acad. Sci. USA 85:8934−8938.
- Segall, J. E., S. M. Block, and H. C. Berg. 1986. Temporal comparisons in bacterial chemotaxis. Proc. Natl. Acad. Sci. USA 83:8987−8991.
- Segall, J. E., A. Ishihara, and H. C. Berg. 1985. Chemotactic signaling in filamentous cells of Escherichia coli. J. Bacteriol. 161:51−59.
- Segall, J. E., M. D. Manson, and H. C. Berg. 1982. Signal processing times in bacterial Chemotaxis. Nature (London) 296:855−857.
- Shapiro, L. 1993. Protein localization and asymmetry in the bacterial cell. Cell 73: 841 855.
- Shepley, D. P., and J. W. Little. 1996. Mutant LexA proteins with specific defects in autodigestion. Proc. Natl. Acad. Sei. USA 93:11 528−11 533.
- Shurvinton, C. E., and R. G. Lloyd. 1982. Damage to DNA induces expression of the ruv gene of Escherichia coli. Mol. Gen. Genet. 185:352−355.
- Siegel, E. 1983. The Escherichia coli uvrD gene is inducible by DNA damage. Mol. Gen. Genet. 191:397−400.
- Siegele, D. A., and Kolter, R. 1992. Life after log. J. Bacterid. 174:345−348.
- Silhavy, T. J., M. L. Berman, and L. W. Enquest. 1984. Experiments with gene fusions. Cold Spring Harbor Laboratory Press, Cold Spring Harbor, New York.
- Slauch, J. M., F. D. Russo, and T. J. Silhavy. 1991. Suppressor mutations in rpoA suggest that OmpR controls transcription by direct interaction with the alpha subunit of RNA polymerase. J. Bacteriol. 173:7501−7510.
- Slauch, J. M., and T. J. Silhavy. 1989. Genetic analysis of the switch that controls porin gene expression in Escherichia coli K-12. J. Mol. Biol. 210:281−292.
- Sledjeski, D., and S. Gottesman. 1995. A small RNA acts as antisilencer of the H-NS-silenced rcsA gene of Escherichia coli. Proc. Natl. Acad. Sei. USA 92:2003−2007.
- Sledjeski, D., A. Gupta, and S. Gottesman. 1996. The small RNA, DsrA, is essential for the low temperature expression of RpoS during exponential growth in Escherichia coli. EMBO J. 15:3993−4000.
- Spassky, A., S. Rimsky, H. Garreau, and H. Buc. 1984. HI a, an E, coli DNA-binding protein which accumulates in stationary phase, strongly compacts DNA in vitro. Nucl. Acids Res. 12:5321−5340.
- Stewart, R. C. 1993. Activating and inhibitory mutations in the regulatory domain of CheB, the methylesterase in bacterial Chemotaxis. J. Biol. Chem. 268:1921−1930.
- Stewart, R. C., A. F. Roth, and F. W. Dahlquist. 1990. Mutations that affect control of the methylesterase activity of CheB, a component of the Chemotaxis adaptation system in Escherichia coli. J. Bacteriol. 172:3388−3399.
- Stock, J. B., G. S. Lukat, and A. M. Stock. 1991. Bacterial Chemotaxis and the molecular logic of intracellular signal transduction networks. Annu. Rev. Biophys. Biophys. Chem. 20:109−136.
- Stock, A. M., J. M. Mottonen, J. B. Stock, and C. E. Schutt. 1989a. Three-dimensional structure of CheY, the response regulator of bacterial Chemotaxis. Nature (London) 337:745−749.
- Stock, J. B., A. J. Ninfa, and A. M. Stock. 19 896. Protein phosphorylation and regulation of adaptive responses in bacteria. Microbiol. Rev. 53:450−490.
- Stock, J. B., A. M. Stock, and J. M. Mottonen. 1990. Signal transduction in bacteria. Nature 344:395−400.
- Stout, V., and S. Gottesman. 1990. RcsB and RcsC: a two-component regulator of capsule synthesis in Escherichia coli. J. Bacteriol. 172:659−669.
- Stout, V., A. Torres-Cabassa, M. R. Maurizi, D. Gutnick, and S. Gottesman. 1991. RcsA, an unstable positive regulator of capsular polysaccharide synthesis. J. Bacteriol. 173:1738−1747.
- Su, W., S. Porter, S. Kustu, and H. Echols. 1990. DNA-looping and enhancer activity: association between DNA-bound NtrC activator and RNA polymerase at the bacterial glnA promoter. Proc. Natl. Acad. Sei. USA 87:5504−5508.
- Swanson, R. V., R. B. Bourret, and M. I. Simon. 1993. Intermolecular complementation of the kinase activity of CheA. Mol. Microbiol. 8:435−441.
- Toman, Z, C. Dambly-Chaudiere, L. Tenenbaum, and M. Radman. 1985. A system for detection of genetic and epigenetic alterations in Escherichia coli induced by DNA-damaging agents. J. Mol. Biol. 186:97−105.
- Torres-Cabassa, A. S., and S. Gottesman. 1987. Capsule synthesis in Escherichia coli K-12 is regulated by proteolysis. J. Bacteriol. 169:981−989.
- Torres-Cabassa, A. S., S. Gottesman, R. D. Frederick, P. J. Dolph, and D. L. Coplin. 1987. Control of extracellular polysaccharide synthesis in Erwinia stewartii and Escherichia coli K-12: a common regulatory function. J. Bacteriol. 169:4525−4531.
- Trisler, P., and S. Gottesman. 1984. Ion transcriptional regulation of genes necessary for capsular polysaccharide synthesis in Escherichia coli K-12. J. Bacteriol. 160:184−191.
- Vieira, J., and J. Messing. 1982. The pUC plasmids, an M13 mp7-derived system for insertion mutagenesis and sequencing with synthetic universal primers. Gene 19:259−268.
- Volz, K., and P. Matsumura. 1991. Crystal structure of Escherichia coli CheY refined at 1.7-A resolution. J. Biol. Chem. 266:15 511−15 519.
- Wada, A., Y. Yamazaki, N. Fujita, and A. Ishihama. 1990. Structure and probable genetic location of a «ribosome modulation factor» associated with 100S ribosomes in stationary-phase Escherichia coli cells. Proc. Natl. Acad. Sei. USA 87:2657−2661.
- Walker, G. C. 1984. Mutagenesis and inducible responses to DNA damage in Escherichia coli. Microbiol. Rev. 48:60−93.
- Walker, G. C. 1985. Inducible DNA repair systems. Annu. Rev. Biochem. 54:425−457.
- Wanner, B. L. 1992. Is cross regulation by phosphorylation of two-component response regulator proteins important in bacteria? J. Bacteriol. 174:2053−2058.
- Way, J. C., M. S. Davis, D. Morisato, D. E. Roberts, and N. Kleckner. 1984. New TnlO derivatives for transposon mutagenesis and for construction of lacZ operon fusions by transposition. Gene 32:369−379.
- Wedel, A., D. S. Weiss, D. Popham, P. Droge, and S. Kustu. 1990. A bacterial enhancer functions to tether a transcriptional activator near a promoter. Science 248:486 490.
- Weiss, D. S., J. Batut, K. E. Klose, J. Keener, and S. Kustu. 1991. The phosphorylated form of the enhancer-binding protein NTRC has an ATPase activity that is essential for activation of transcription. Cell 67:155−167.
- Weiss, D. S., F. Claverie-Martin, and B. Magasanik. 1992. Phosphorylation of nitrogen regulator I of Escherichia coli induces strong cooperative binding to DNA essential for activating of transcription. Proc. Natl. Acad. Sei. USA 89:5088−5092.
- Weiss, D. S., and B. Magasanik. 1988. Phosphorylation of nitrogen regulator I (NRi) of Escherichia coli. Proc. Natl. Acad. Sei. USA 85:8919−8923.
- Whittier, R. F., and J. W. Chase. 1983. DNA repair properties of Escherichia coli tif-1, recAo281 and lexAl strains deficient in single-strand DNA binding protein. Mol. Gen. Genet. 190:101−111.
- Witkin, E. M. 1976. Ultraviolet mutagenesis and inducible deoxyribonucleic acid repair in Escherichia coli. Bacteriol. Rev. 40:869−707.
- Wolfe, A. J., and R. Stewart. 1993. The short form of the Che A protein restores kinase activity and chemotactic ability to kinase-deficient mutants. Proc. Natl. Acad. Sei. USA 90:1518−1522.
- Wootton, J. C., and M. H. Drummond. 1989. The Q-linker: a class of interdomain sequences found in bacterial multidomain regulatory proteins. Protein Eng. 2:535−543.
- Wylie, D., A. M. Stock, C. Y. Wong, and J. B. Stock. 1988. Sensory transduction in bacterial Chemotaxis involves phosphotransfer between Che proteins. Biochem. Biophys. Res. Commun. 151:891−896.
- Yang, Y., and M. Inouye. 1991. Intermolecular complementation between two defective mutants of Escherichia coli signal-transducing receptors. Proc. Natl. Acad. Sei. USA 88:11 057−11 061.
- Yim, H. H., and M. Villarejo. 1992. osmY, a new hyperosmotically inducible gene, encodes a periplasmic protein in Escherichia coli. J. Bacteriol. 174:3637−3644.
- Yura, T., H. Nagai, and H. Mori. 1993. Regulation of heat-shock response in bacteria. Annu. Rev. Microbiol. 47:321−350.
- Zaug, A. J., and T. R. Cech. 1986. The intervening sequence RNA of Tetrahymena is an enzyme. Science 231:470−475.
- Zinkevich-Peotti, K., and J. M. Fraser. 1988. New locus for exopolysaccharide overproduction in Escherichia coli K-12. J. Bacteriol. 170:1405−1407.