Пептидные производные 5-О-микаминозилтилонолида как инструменты для изучения рибосомного туннеля
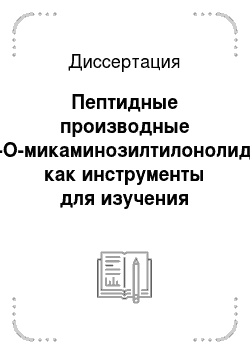
Диссертация
С помощью компьютерного моделирования на основе пептидных производных 5-О-микаминозилтилонолида осуществлен дизайн потенциальных зондов, которые могут быть использованы как инструменты для изучения регуляторного района рибосомного туннеля. Осуществлен синтез серии новых конъюгатов 5-О-микаминозилтилонолида по положению С23 с пептидами, в том числе содержащими остатки фенилаланина и триптофана… Читать ещё >
Содержание
- Список сокращений
- Глава 1. Растущий пептид в рибосомном туннеле (Обзор литературы)
- 1. 1. Строение и функционирование рибосомы
- 1. 1. 1. Морфология бактериальной рибосомы
- 1. 1. 2. Функционирование рибосомы
- 1. 2. Рибосомный туннель
- 1. 1. Строение и функционирование рибосомы
- Сайт связывания макролидных антибиотиков
- 1. 3. Взаимодействия растущих пептидов со стенками рибосомного туннеля
- 1. 3. 1. Экспрессия генов cat и clmA
- 1. 3. 2. Регуляция экспрессии гена secA
- 1. 3. 3. Регуляция трансляции белка YbeL
- 1. 3. 4. Триптофаназный оперон. Механизм регуляции экспрессии
- 1. 3. Взаимодействия растущих пептидов со стенками рибосомного туннеля
- 2. 1. Дизайн пептидных производных ОМТ как инструментов для изучения функционирования РТ
- 2. 2. Получение антибиотика 5-О-микаминозилтилонолида
- 2. 3. Разработка метода синтеза аминокислотных и пептидных производных ОМТ по положению С
- 2. 4. Синтез N-защищенных пептидов, содержащих остатки фенилаланина и триптофана
- 2. 5. Синтез фенилаланин- и триптофан-содержащих пептидных производных ОМТ по положению С
- 2. 6. Изучение биологической активности пептидных производных ОМТ по положению
- 2. 6. 1. Изучение связывания ОМТ и его производных с рибосомой
- 2. 6. 2. Исследование ингибирующей активности производных ОМТ в системе транскрипции-трансляции зеленого флуоресцентного белка GFP
- 2. 6. 3. Исследование ингибирующей активности производных ОМТ в системе трансляции мРНК люциферазы светлячков
- 2. 6. 4. Изучение антибиотической активности триптофан-содержащих производных ОМТ
- 3. 1. Исходные вещества и очистка растворителей
- 3. 1. 1. Исходные вещества
- 3. 1. 2. Абсолютирование растворителей
- 3. 2. Вспомогательные методы
- 3. 2. 1. Хроматография в тонком слое
- 3. 2. 2. Препаративная тонкослойная хроматография
- 3. 2. 3. Колоночная хроматография
- 3. 2. 4. Высокоэффективная жидкостная хроматография
- 3. 2. 5. Гидролиз пептидов и аминокислотный анализ
- 3. 2. 6. Масс-спектрометрия
- 3. 2. 7. ЯМР-спектроскопия
- 3. 3. Методики получения веществ
- 3. 4. Методики биологических испытаний
- 3. 4. 1. Буферы и растворы
- 3. 4. 2. Выделение рибосом Е. col
- 3. 4. 3. Химический пробинг
- 3. 4. 4. Конкурентное вытеснение радиоактивно меченого [14С]-эритромицина
- 3. 4. 5. Система транскрипции-трансляции зеленого флуоресцентного белка GFP
- 3. 4. 6. Система трансляции мРНК люциферазы светлячков
Список литературы
- Nissen P., Hansen J., Ban N., Moore P.B., Steitz T.A. The structural basis of ribosome activity in peptide bond synthesis. Science (2000) 289, 920−930.
- Богданов A.A. О некоторых структурных аспектах пептидилтрансферазной реакции. Мол. Биол. (2003) 37, 511−515.
- Tenson Т., Ehrenberg М. Regulatory nascent peptides in the ribosomal tunnel. Cell (2002) 108, 591−594.
- Сумбатян H.B., Коршунова Г. А., Богданов A.A. Пептидные производные ингибиторов синтеза белка антибиотиков тилозина и десмикозина. Биохимия (2003) 68, 1436−1438.
- Lake J.A. Ribosome structure determined by electron microscopy of Escherichia coli small subunits, large subunits and monomeric ribosomes. J. Mol. Biol. (1976) 105, 131−159.
- Stoffler G., Stoffler-Meilicke, M. Immunoelectron microscopy of ribosomes. Annu. Rev. Biophys. Bioeng. (1984) 13, 303−330.
- Frank J. Image analysis of single macromolecules. Electron. Microsc. Rev. (1989) 2, 53−74.
- Stark H., Mueller F., Orlova E.V., Schatz M., Dube P., Erdemir Т., Zemlin F., Brimacombe R., van Heel M. The 70S Escherichia coli ribosome at 23 A resolution: fitting the ribosomal RNA. Structure (1995) 3, 815−821.
- Bernstein F.C., Koetzle T.F., Williams G.J., Meyer E.F.J., Brice M.D., Rodgers J.R., Kennard O., Shimanouchi Т., Tasumi M. The Protein Data Bank: a computerbased archival file for macromolecular structures. J. Mol. Biol. (1977) 112, 535−542.
- Trakhanov S., Yusupov M., Shirokov V., Garber M., Mitschler A., Ruff M., Thierry J.C., Moras D. Preliminary X-ray investigation of 70 S ribosome crystals from Thermus thermophilic. J. Mol. Biol (1989) 209, 327−328.
- Yusupov M., Garber M.B., Vasiliev V.D., Spirin A.S. Thermus thermophilics ribosomes for crystallographic studies. Biochimie (1991) 73, 887−897.
- Ban N., Nissen P., Hansen J., Moore P.B., Steitz T.A. The complete atomic structure of the large ribosomal subunit at 2.4 A resolution. Science (2000) 289, 905−920.
- Schluenzen F., Tocilj A., Zarivach R., Harms J., Gluehmann M., Janell D., Bashan A., Bartels H., Agmon I., Franceschi F., Yonath A. Structure of functionally activated small ribosomal subunit at 3.3 angstroms resolution. Cell (2000) 102, 615−623.
- Yusupov M.M., Yusupova G.Z., Baucom A., Lieberman K., Earnest T.N., Cate J.H., Noller H.F. Crystal structure of the ribosome at 5.5 A resolution. Science (2001)292, 883−896.
- Shatsky I., Evstafieva A.G., Bystrova T.F., Bogdanov A.A., Vasiliev V.D. Topography of RNA in the ribosome: location of the З'-end of 5S RNA on the central protuberance of the 50S subunit. FEBS Lett. (1980) 121, 97−100.
- Bernabeu C., Lake J.A. Nascent polypeptide chains emerge from the exit domain of the large ribosomal subunit: immune mapping of the nascent chain. Proc. Natl. Acad. Sci. USA (1982) 79, 3111−3115.
- Bernabeu C., Tobin E.M., Fowler A., Zabin I., Lake J.A. Nascent polypeptide chains exit the ribosome in the same relative position in both eucaryotes and procaryotes. J. Cell. Biol. (1983) 96, 1471−1474.
- Milligan R.A., Unwin P.N. Location of exit channel for nascent protein in 80S ribosome. Nature (1986) 319, 693−695.
- Yonath A., Leonard K.R., Wittmann H.G. A tunnel in the large ribosomal subunit revealed by three-dimensional image reconstruction. Science (1987) 236, 813 816.
- Stark H., Orlova E.V., Rinke-Appel J., Junke N., Mueller F., Rodnina M., Wintermeyer W., Brimacombe R., van Heel M. Arrangement of tRNAs in pre- and posttranslocational ribosomes revealed by electron cryomicroscopy. Cell1997) 88, 19−28.
- Stark H., Rodnina M.V., Rinke-Appel J., Brimacombe R., Wintermeyer W., Van-Heel M. Visualization of elongation factor Tu on the Escherichia coli ribosome. Nature (1997) 389, 403−406.
- Agrawal R.K., Penczek P., Grassucci R.A., Li Y.H., Leith A., Nierhaus K.H., Frank J. Direct visualization of A-, P-, and E-site transfer RNAs in the Escherichia coli ribosome. Science (1996) 271, 1000−1002.
- Agrawal R.K., Penczek P., Grassucci R.A., Frank J. Visualization of elongation factor G on the Escherichia coli 70S ribosome: the mechanism of translocation. Proc. Natl. Acad. Sci. USA (1998) 95, 6134−6138.
- Schmeing T.M., Ramakrishnan V. What recent ribosome structures have revealed about the mechanism of translation. Nature (2009) 461, 1234−1242.
- Samaha R.R., Green R., Noller H.F. A base pair between tRNA and 23S rRNA in the peptidyl transferase centre of the ribosome. Nature (1995) 377, 309−314.
- Green R., Switzer C., Noller H.F. Ribosome-catalyzed peptide-bond formation with an A-site substrate covalently linked to 23 S ribosomal RNA. Science1998) 280,286−289.
- Hansen J.L., Schmeing T.M., Moore, P.B., Steitz, A.T. Structural insight into peptide bond formation. Proc. Natl. Acad. Sci. USA (2002) 99, 11 670−11 675.
- Green R., Noller H.F. Ribosomes and translation. Annu. Rev. Biochem. (1997) 66, 679−716.
- Moore P.B.- Steitz T.A. The structural basis of large ribosomal subunit function. Annu. Rev. Biochem. (2003) 72, 813−850.
- Valle M., Zavialov A., Sengupta J., Rawat U., Ehrenberg M., Frank J. Locking and unlocking of ribosomal motions. Cell (2003) 114, 123−134.
- Ogle J.M., Brodersen D.E., Clemons W.M., Tany M.J., Carter A.P., Ramakrishnan V. Recognition of cognate transferRNA by the 3OS ribosomal subunit. Science (2001) 292, 897−902.
- Ogle J.M., Murphy F.V., Tarry M.J., Ramakrishnan V. Selection of tRNA by the ribosome requires a transition from an open to a closed form. Cell (2002) 111, 721 732.
- Sievers A., Beringer M., Rodnina M. V., Wolfenden R. The ribosome as an entropy trap. Proc. Natl. Acad. Sci. USA (2004) 101, 7897−7901.
- Schmeing T.M., Huang K. S., Strobel S. A., Steitz, T. A. An induced-fit mechanism to promote peptide bond formation and exclude hydrolysis of peptidyl-tRNA. Nature (2005) 438, 520−524.
- Jenni S., Ban N. The chemistry of protein synthesis and voyage through the ribosomal tunnel. Cur. Opin. Struct. Biol. (2003) 13, 212−219.
- Polacek N., Gaynor M., Yassin A., Mankin A. S. Ribosomal peptidyl transferase can withstand mutations at the putative catalytic nucleotide. Nature (2001) 411, 498−501.
- Maguire B.A., Beniaminov A. D., Ramu H., Mankin A. S.} Zimmermann R. A. A protein component at the heart of an RNA machine: the importance of protein L27 for the function of the bacterial ribosome. Mol. Cell (2005) 20, 427−435.
- Moore V.G., Atchison R. E., Thomas G., Moran M., Noller H. F. Identification of a ribosomal protein essential for peptidyl transferase activity. Proc. Natl. Acad. Sci. USA (1975) 72, 844−848.
- Trobro S., Aqvist J. Role of ribosomal protein L27 in peptidyl transfer. Biochemistry (2008) 47, 4898−4906.
- Voorhees R.M., Weixlbaumer A., Loakes D., Kelley A. C., Ramakrishnan, V. Insights into substrate stabilization from snapshots of the peptidyl transferase center of the intact 70S ribosome. Nat. Struct. Mol. Biol. (2009) 16, 528−533.
- Moore P.B. The ribosome at atomic resolution. Biochemistry (2001) 40 32 433 250.
- Voss N.R., Gerstein M., Steitz T.A., Moore P.B. The geometry of the ribosomal polypeptide exit tunnel. J. Mol. Biol. (2006) 360, 893−906.
- Frank J., Zhu J., Penczek P., Li Y., Srivastava S., Verschoor A., Radermacher M., Grassucci R., Lata R.K., Agrawal R.K. A model of protein synthesis based on cryo-electron microscopy of the E. coli ribosome. Nature (1995) 376, 441−444.
- Pool M.R., Stumm J., Fulga T.A., Sinning I., Dobberstein B. Distinct modes of signal recognition particle interaction with the ribosome. Science (2002) 297, 13 451 348.
- Keenan R.J., Freymann D.M., Stroud R.M., Walter P. The signal recognition particle. Annu. Rev. Biochem. (2001) 70, 755−775.
- Gong F., Yanofsky C. Instruction of translating ribosome by nascent peptide. Science (2002)297, 1864−1867.
- Frank J., Agrawal R.K. A ratchet-like inter-subunit reorganization of the ribosome during translocation. Nature (2000) 406, 318−322.
- Gabasvili I.S., Gregory S.T., Valle M., Grassucci R., Worbs M., Wahl M.C., Dahlberg A.E., Frank J. The polypeptide tunnel system in the ribosome and its gating in erythromycin resistance mutants of L4 and L22. Mol. Cell (2001) 8, 181−188.
- Hansen J.L., Ban N., Nissen P., Moore P.B., Steitz T.A. The structures of four macrolide antibiotics bound to the large ribosomal subunit. Mol. Cell (2002) 10, 117−126.
- Auerbach Т., Bashan A., Yonath A. Ribosomal antibiotics: structural basis for resistance, synergism and selectivity. Trends Biotech. (2004) 22, 570−576.
- Streitz Т. On the structural basis of peptide-bond formation and antibiotic resistance from atomic structures of the large ribosomal subunit. FEBS Lett. (2005) 579, 955−958.
- Poulsen S.M., Kofoed C., Veater B. Inhibition of the ribosomal peptidyl transferase reaction by the mycarose moiety of the antibiotics carbomycin, spiramycin and tylosin. J. Mol. Biol. (2000) 304, 471−481.
- Mankin A. Macrolide myths. Cur. Opin. Microbiol. (2008) 11, 414−421.
- Nakatogawa H., Ito K. The ribosomal exit tunnel functions as a discriminating gate. Cell (2002) 108, 629−636.
- Vazquez-Laslop N., Thum C., Mankin, A.S. Molecular mechanism of drug-dependent ribosome stalling. Mol. Cell (2008) 30, 190−202.
- Ramu H., Mankin A., Vazquez-Laslop N. Programmed drug-dependent ribosome stalling. Mol. Microbiol. (2009) 71, 811−824.
- Lovett P. S. Nascent peptide regulation of translation. J. Bacteriol. (1994) 176, 6415−6417.
- Lovett P. S., Rogers E.J. Ribosome regulation by the nascent peptide. Microbiol. Rev. (1996) 60, 366−385.
- Gu Z., Harrod R., Rogers E.J., Lovett P. S. Anti-peptidyltransferase leader peptides of attenuation-regulated chloramphenicol-resistance genes. Proc. Natl. Acad. Sci. USA (1994) 91, 5612−5616.
- Rogers E.J., Lovett P. S. The cis-effect of a nascent peptide on its translating ribosome: influence of the cat-86 leader peptide on translation termination at leader codon 6. Mol. Microbiol. (1994) 12, 181−186.
- Nakatogawa H., Ito К. Intraribosomal regulation of expression and fate of proteins. ChemBioChem (2004) 5, 48−51.
- Muto H., Nakatogawa H., Ito K. Genetically encoded but nonpolypeptide prolyl-tRNA functions in the A site for SecM-mediated ribosomal stall. Mol. Cell (2006) 22, 545−552.
- Woolhead C.A., Johnson A.E., Bernstein H.D. Translation arrest requires two-way communication between a nascent polypeptide and the ribosome. Mol. Cell (2006) 22, 587−598.
- Suhonara Т., Abo Т., Inada Т., Aiba H. The C-terminal amino acid sequence of nascent peptide is a major determinant of SsrA tagging at all three stop codons. RNA (2002) 8, 1416−1427.
- Roche E.D., Sauer R.T. Identification of endogenous SsrA-tagged proteins reveals tagging at positions corresponding to stop codons. J. Biol. Chem. (2001) 276, 28 509−28 515.
- Wang D., Ding X., Rather P.N. Indole can act as an extracellular signal in Escherichia coli. J. Bacteriol. (2001) 183, 4210−4216.
- Ren D., Bedzyk L.A., Ye R.W., Thomas S.M., Wood Т.К. Stationary-phase quorum-sensing signals affect autoinducer-2 and gene expression in Escherichia coli. Appl. Environ. Microbiol. (2004) 70, 2038−2043.
- Hirakawa H., Inazumi Y., Masaki Т., Hirata Т., Yamaguchi A. Indole induces the expression of multidrug exporter genes in Escherichia coli. Mol. Microbiol. (2005) 55, 1113−1126.
- Kazarinoff M.N., Snell E.E. Essential arginine residues in triptophanase from Escherichia coli. J. Biol. Chem. (1977) 252, 7598−7602.
- Gollnick P., Yanofsky C. tRNATrp translation of leader peptide codon 12 and other factors that regulate expression of the tryptophanase operon. J. Bacteriol. (1990) 172,3100−3107.
- Gong F., Yanofsky C. Reproducing tna operon regulation in vitro in an S-30 system. Tryptophan induction inhibits cleavage of TnaC peptidyl-tRNA. J. Biol. Chem. (2001)276, 1974−1983.
- Yanofsky C. RNA-based regulation of genes of tryptophan synthesis and degradation, in bacteria. RNA (2007) 13, 1141−1154.
- Gong F., Ito K., Nakamura Y., Yanofsky C. The mechanism of tryptophan induction of tryptophanase operon expression: tryptophan inhibits release factor-mediated cleavage of TnaC-peptidyl-tRNA (Pro). Proc. Natl. Acad. Sci. USA (2001) 98, 8997−9001.
- Gong M., Cruz-Vera L.R., Yanofsky, C. RRF and RF3 action promotes TnaC-peptidyl-tRNA dropoff and relieves ribosome stalling during tryptophan induction of tna operon expression in Escherichia coli. J. Bacteriol. (2007) 189, 3147−3155.
- Cruz-Vera L.R., Rajagopal S., Squires C., Yanofsky C. Features of ribosome-peptidyl-tRNA interactions essential for tryptophan induction of tna operon expression. Mol. Cell (2005) 19, 333−343.
- Cruz-Vera L.R., New A., Squires C., Yanofsky, C. Ribosomal features essential for tna operon induction: tryptophan binding at the peptidyl transferase center. J. Bacteriol. (2007) 189, 3140−3146.
- Mankin A.S. Nascent peptide in the l3irth canal' of the ribosome. Trends Biochem. Sci. (2006)31, 11−13.
- Gong M., Gong F., Yanofsky, C. Overexpression of tnaC of Escherichia coli inhibits growth by depleting tRNA2Pro availability. J. Bacteriol. (2006) 188, 1892−1898.
- Omura S. Macrolide antibiotics: chemistry, biology and practice. Academic Press: Orlando, FL (2002).
- Karahalios P., Kalpaxis D.L., Fu H., Katz L., Wilson D.N., Dinos G.P. On the mechanism of action of 9-O-arylalkyloxime derivatives of 5-O-mycaminosyltylonolide, a new class of 16-membered macrolide antibiotics. Mol. Pharmacol (2006) 70, 1271−1280.
- Gaynor M., Mankin A.S. Macrolide antibiotics: binding site, mechanism of action, resistance. Curr. Top. Med. Chem. (2003) 3, 949−961.
- Yusupova G., Jenner L., Rees В., Moras D., Yusupov M. Structural basis for messenger RNA movement on the ribosome. Nature (2006) 444, 391−394.
- Brodersen J.E., Clemons W.M.Jr., Carter A.P., Morgan-Warren R.J., Wimberley B.T., Ramakrishnan V. The structural basis for the action of the antibiotics tertacyclin, pactpmycin and hygromycin В on 30S ribosomal subunit. Cell (2000) 103, 1143−1154.
- Wilson D.N., Harms J., Nierhaus K.H., Schluenzen F., Fucini P. Species-specific antibiotic-ribosome interactions: implications for drug development. Biol Chem. (2005)386, 1239−1252.
- Morin R., Gorman M. O-Mycaminosiltylonolide and process for the preparation thereof (1969). US Patent № 3 459 853.
- Kirst H., Toth J. С-23-Modified derivatives of OMT (1984). GB Patent № 2 123 828.
- Jian Т., Phanly Т., Busuyek M" Hou Y., Or Y., Qiu Y., Vo N. 23−0-Substituted 5-O-mycaminosyltylonolide derivatives (2003). US Patent № 675 3415B2.
- Fujivvara Т., Watanabe H., Hirano Т., Sakakibara H. 23−0-Acyl-23-demycinosyldesmycosin derivatives (1983). GB Patent № 2 116 170 A.
- Sheehan J.C., Hess G.P. A new method of forming peptide bonds. J. Am. Chem. Soc. (1955) 77, 1067−1068.
- Wang S.S., Tam J.P., Wang B.S.H., Merrifield R.B. Enchancement of peptide-coupling reactions by 4-dimethylaminopyridine. Int. J. Protein Res. (1981) 18, 459−467.
- Atherton E., Benoiton N.L., Brown E., Sheppard R.C., Williams B.J. Racemization of activated, urethane-protected amino-acids by /?-dimethylaminopyridine. Significance in solid-phase peptide synthesis. J. Chem. Soc., Chem. Commun. (1981) 7, 336−337.
- Xu S., Held I., Kempf В., Mayr H., Steglich W., Zipse H. The DMAP-catalized acetylation of alcohols a mechanistic study (DMAP = 4-(dimethylamino)pyridine). Chem. Eur. J. (2005) 11, 4751−4757.
- Kirst H.A., Toth J.E. Method of preparing 23-monoesters of OMT and DMT (1984). US Patent № 4 487 923.
- Wunsch E. Houben-Weyl on peptides and peptidomimetics. Thieme: Shtuttgart-New York (1974) — Vol. 15/1.
- Guttman S., Pless J., Boissonas R.A. Nouvelle synthese de la bradykinine. Helv. Chim. acta (1962) 45, 170−177
- Anderson G.W., McGregor A.C. f-Butyloxycarbonylamino acids and their use in peptide synthesis. J. Am. Chem. Soc. (1957) 79, 6180−6183.
- Konig W., Geiger R. Eine neue Methode zur Synthese von Peptiden: Aktivierung der Carboxylgruppe mit Dicyclohexylcarbodiimid unter Zusatz von 1-Hydroxy-benzotriazolen. Chem. Ber. (1970) 103, 788−798.
- Carpino L.A., El-Faham A. Effect of tertiary bases on O-benzotriazolyluronium salt-induced peptide segment coupling. J. Org. Chem. (1994) 59, 695−698.
- Brenner M., Huber W. Herstellung von a-Aminosaureestern durch Alkoholyse der Methyl ester. Helv. Chim. acta (1953) 36.
- Castro В., Dormoy J.R., Evin G., Selve C. Reactifs de couplage peptidique I (l)-l'hexafluorophosphate de benzotriazolyl N-oxytrisdimethylamino phosphonium (B.O.P.). Tetrahedron Lett. (1975) 16, 1219−1222.
- Stern S., Moazed D., Noller H.F. Structural analysis of RNA using chemical and enzymatic probing monitored by primer extension. Methods Enzymol. (1988) 164, 481−489.
- Petropoulos A.D., Kouvela E.C., Dinos G.P., Kalpaxis D.L. Stepwise binding of tylosin and erythromycin to Escherichia coli ribosomes, characterized by kinetic and footprinting analysis. J. Biol. Chem. (2008) 283, 4756−4765.
- Титце JL, Айхер Т. Препаративная органическая химия. Реакции и синтез в практикуме органической химии и научно-исследовательской лаборатории. Мир: Москва (1999).
- Zubay G. In vitro synthesis of protein in microbial systems. Annu. Rev. Genet. (1973)7, 267−287.
- Kolb V.A., Makeyev E.V., Spirin A.S. Co-translational folding of an eukaryotic multidomain protein in a prokaryotic translation system. J. Biol. Chem. (2000) 275, 16 597−16 601.
- Svetlov M.S., Kommer A., Kolb V.A., Spirin, A.S. Effective cotranslational folding of firefly luciferase without chaperones of the Hsp70 family. Protein Science (2006) 15, 242−247.