Получение генетически кодируемых FRET-сенсоров каспазы-3 на основе тербий-связывающего пептида и красных флуоресцентных белков DsRed2 и TagRFP
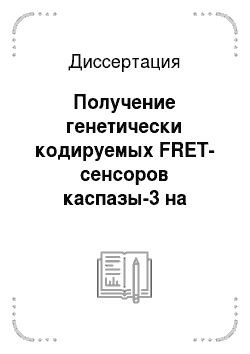
Диссертация
Тем не менее, проблема фонового сигнала клеток, связанная с автофлуоресценцией биомолекул и светорассеянием остается открытой. Для решения этой проблемы разрабатывают сенсоры на основе белков со спектром флуоресценции в красной и дальнекрасной области. Другим подходом является использование в качестве доноров во БИЕТ-паре флуоресцирующих комплексов лантанидов с микрои миллисекундным временем… Читать ещё >
Содержание
- СПИСОК СОКРАЩЕНИЙ
- ГЛАВА 1. ОБЗОР ЛИТЕРАТУРЫ
- 1. 1. Изучение ферментативной активности каспазы-3 с помощью FRET-биосенсоров
- 1. 2. Проблема фоновой флуоресценции
- 1. 3. Флуоресцентные свойства лантанидов. Сенсибилизация флуоресценции. Лантанид-связывающие пептиды
- 1. 4. Многофотонное возбуждение лантанидов
- 1. 5. Основные тенденции изучения индуктивно-резонансного переноса энергии
- 1. 6. Комплексные соединения лантанидов в качестве доноров во FRET-парах при изучении биологических процессов и проведении биоаналитических реакций
- ГЛАВА 2. МАТЕРИАЛЫ И МЕТОДЫ
- 2. 1. Оборудование
- 2. 2. Реактивы
- 2. 3. Методы исследования
- ГЛАВА 3. РЕЗУЛЬТАТЫ И ОБСУЖДЕНИЕ
- 3. 1. Характеристика экспрессии флуоресцентных белков в условиях in vitro и in vivo
- 3. 2. Получение генетически кодируемых конструкций, кодирующих гибридные белки. г
- 3. 3. Получение высокоочищенных фракций гибридных белков и определение их агрегатного состояния
- 3. 4. Изучение переноса энергии
- 3. 5. Определение активности каспазы-З
Список литературы
- Mazumder S., Plesca D., Almasan A. Caspase-3 activation is a critical determinant of genotoxic stress-induced apoptosis. Methods Mol. Biol., 2008, v. 414, pp. 13−21.
- Meier P., Finch A., Evan G. Apoptosis in development. Nature, 2000, v. 407, pp. 796−801.
- Forster Th. Delocalized Excitation and Excitation Transfer. -Modern Quantum Chemistry, ed. by Sinanoglu O. N.Y.: Academic Press, 1965, pp. 93−138.
- Vogel S.S., Thaler C., Koushik S.V. Fanciful FRET. Sei. STKE, 2006,1. 331, re2.
- Li I.T., Pham E., Truong K. Protein biosensors based on the principle of fluorescence resonance energy transfer for monitoring cellular dynamics. Biotechnol .Lett., 2006, v. 28, pp. 1971−1982.
- Piston D.W., Kremers G.-J. Fluorescent protein FRET: the good, the bad and the ugly. -Trends Biochem. Sei., 2007, v. 32, № 9, pp. 407−414.
- Karasawa S., Araki T., Nagai T., Mizuno H., Miyawaki A. Cyan-emitting and orange-emitting fluorescent proteins as a donor/ acceptor pair for fluorescence resonance energy transfer. -Biochem. J., 2004, v. 381, pp. 307−312.
- Wu X., Simone J., Hewgill D., Siegel R., Lipsky P.E., He L. Measurement of Two Caspase Activities Simultaneously in Living Cells by a Novel Dual FRET Fluorescent Indicator Probe. -Cytometry Part A, 2006, v. 69A, pp. 477−486.
- Shcherbo D., Souslova E. A, Goedhart J., Chepurnykh T. V, Gaintzeva A., Shemiakina I. l, Gadella T. WJ, Lukyanov S., Chudakov D.M. Practical and reliable FRET/FLIM pair of fluorescent proteins. BMC Biotechnology, 2009, v. 9, i. 24, pp. 1−6.
- Hemmil’a I., Laitala V. Progress in Lanthanides as Luminescent Probes. J. Fluoresc., 2005, v. 15, № 4, pp. 529−542.
- Horton R.A., Strachan E.A., Vogel K.W., Riddle S.M. A substrate for deubiquitinating enzymes based on time-resolved fluorescence resonance energy transfer between terbium and yellow fluorescent protein. Anal. Biochem., 2007, v. 360, i. 1, pp. 138−143.
- Thornberry N.A., Rano T.A., Peterson E.P., Rasperi D.M., Timkey T., Garcia-Calvo M.,
- Houtzageri V.M., Nordstromi P.A., Royi S., Vaillancourti J.P., Chapman K.T., Nicholsoni D.W.
- A combinatorial approach defines specificities of members of the caspase family and granzyme100
- B. Functional relationships established for key mediators of apoptosis. J. Biol. Chem., 1997, v. 272, i. 29, pp. 17 907−17 911.
- Hoeppner D.J., Hengartner M.O., Schnabel R. Engulfment genes cooperate with ced-3 to promote cell death in Caenorhabditis elegans. Nature, 2001, v. 412, pp. 202−206.
- Nicholson D. W, ICE/CED3-like proteases as therapeutic targets for the control of inappropriate apoptosis. Nat. Biotechnol., 1996, v. 14, pp. 297−301.
- Blankenberg F.G. Monitoring of treatment-induced apoptosis in oncology with PET and SPECT. Curr. Pharm. Des., 2008, v. 14, pp. 2974−2982.
- Tyas L., Brophy V.A., Pope A., Rivett A.J., Tavare J.M. Rapid caspase-3 activation during apoptosis revealed using fluorescence-resonance energy transfer. EMBO, 2000, v. 1, pp. 266 270.
- Morgan M.J., Thorburn A. Measurement of caspase activity in individual cells reveals differences in the kinetics of caspase activation between cells. Cell Death Differ., 2001, v. 8, pp. 38−43.
- Chiang J. J, Truong K. Using co-cultures expressing fluorescence resonance energy transfer based protein biosensors to simultaneously image caspase-3 and Ca2+ signaling. Biotechnol. Lett., 2005, v. 27, № 16, pp. 1219−1227.
- Luo K.Q., Yu V.C., Pu Y., Chang D.C. Measuring dynamics of caspase-8 activation in a single living HeLa cell during TNFalpha-induced apoptosis. Biochem. Biophys. Res. Commun., 2003, v. 304, № 2, pp. 217−222.
- Tyas L., Brophy V.A., Pope A., Rivett A.J. and Tavare M. Rapid caspase-3 activation during apoptosis revealed using fluorescence-resonanse energy transfer. EMBO Reports, 2000, v. 1, i. 3, pp. 266−270.
- Lin J., Zhang Z., Yang J., Zeng S., Liu B.F., Luo Q. Real-time detection of caspase-2 activation in a single living HeLa cell during cisplatin-induced apoptosis. J. Biomed. Opt., 2006, v. 11, i. 2, 24 011.
- Mahajan N.P., Harrison-Shostak D.C., Michaux J., Herman B. Novel mutant green fluorescent protein protease substrates reveal the activation of specific caspases during apoptosis. Chem. Biol., 1999, v. 6, i. 6, pp. 401−409.
- Xu X., Gerard A.L.V., Huang Betty C.B., Anderson D.C., Payan D.G., Luo Y. Detection of programmed cell death using fluorescence energy transfer. Nucl. Acids Res., 1998, v. 26, № 8, pp. 2034−2035
- Angres B., Steuer H., Weber P., Wagner M., Schneckenburger H. A Membrane-Bound FRET-Based Caspase Sensor for Detection of Apoptosis Using Fluorescence Lifetime and Total Internal Reflection Microscopy. Cytometry Part A, 2009, v. 75A, pp. 420−427.
- Takemoto K., Nagai T., Miyawaki A., Miura M. Spatio-temporal activation of caspase revealed by indicator that is insensitive to environmental effects. — J. Cell Biol., 2003, v. 160. i. 2, pp. 235−243.
- Nagai T., Miyawaki A. A high-throughput method for development of FRET based indicators for proteolysis. Biochem. Biophys. Res. Commun., 2004, v. 319, pp. 72−77.
- Xu X., Gerard A. L V., Huang B.C.B., Anderson D.C., Payan D.G., Luo Y. Detection of programmed cell death using fluorescence energy transfer. Nucl. Acids Res., 1998, v. 26, pp. 2034−2035.
- Wang F., Chen T.-S., Xing D., Wang J.-J., Wu Y.-X. Measuring Dynamics of Caspase-3 Activity in Living Cells Using FRET Technique During Apoptosis Induced by High Fluence Low-Power Laser Irradiation. Lasers in Surgery and Medicine, 2005, v. 36, pp. 2−7.
- Billinton N., Knight A.W. Seeing the Wood through the Trees: A Review of Techniques for Distinguishing Green Fluorescent Protein from Endogenous Autofluorescence. Anal. Biochem., 2001, v. 291, pp. 175−197.
- Monici M. Cell and tissue autofluorescence research and diagnostic applications. -Biotechnol. Annu. Rev., 2005, v. 11, pp. 227−256.
- Pawley J.B. Handbook of Biological Confocal Microscopy, third edition. New York: Springer Science Business Media, 2006.
- Yuan J., Wang G. Lanthanide Complex-Based Fluorescence Label for Time-Resolved Fluorescence Bioassay. J. Fluoresc., 2005, v. 15, № 4, pp. 559−568.
- Corrodi H., Jonsson G. The Formaldehyde Fluorescence Method for the Histochemical Demonstration of Biogenic Monoamines. J. Histochem. Cytochem., 1967, v. 15, № 2, pp. 6578.
- Levenson R.M., Mansfield J.R. Multispectral Imaging in Biology and Medicine. Cytometry A., 2006, v. 69, pp. 748−758.
- Aubin J.E. Autofluorescence of Viable Cultured Mammalian Cells. J. Histochem. Cytochem., 1979, v. 27, № 1, pp. 36−43.
- Nokubo M., Ohta M., Kitani K., Nagy I. Identification of protein-bound riboflavin in rat hepatocyte plasma membrane as a source of autofluorescence. Biochim. Biophys. Acta., 1989, v. 981, i. 2, pp. 303−308.
- Benson R.C., Meyer R.A., Zaruba M.E., McKhann G.M. Cellular Autofluorescence Is It Due To Flavins? — J. Histochem. Cytochem., 1979, v. 27, № 1, pp. 44−48.
- Sewell W.F., Mroz E.A. Flavin adenine dinucleotide is a major endogenous fluorophore in the inner ear. Hear Res., 1993, v. 70, i. 2, pp. 131−138.
- Galeotti T., van Rossum G.D., Mayer D.H., Chance B. On the fluorescence of NAD (P)H in whole-cell preparations of tumours and normal tissues. — Eur. J. Biochem., 1970, v. 17, i. 3, pp. 485−496.
- Schneckenburger H., Gessler P., Pavenstadt-Grupp I. Measurements of Mitochondrial Deficiencies in Living Cells by Microspectrofluorometry. J. Histochem. Cytochem., 1992, v. 40, № 10, pp. 1573−1578.
- Gao G., Ollinger K, Brunk U T. Influence of intracellular glutathione concentration of lipofuscin accumulation in cultured neonatal rat cardiac myocytes. Free Radie. Biol. Med., 1994, v. 16, i. 2, pp. 187−194.
- Wolman M. Lipid pigments (chromolipids): their origin, nature, and significant J. Pathobiol., 1980, v. 10, pp. 253−267.
- Dowson J.H. The evaluation of autofluorescence emission spectra derived from neuronal lipopigment. J. Microsc., 1982, v. 128, pp. 261−270.
- Yin D., Yuan X., Brunk U.T. Test-tube simulated lipofuscinogenesis. Effect of oxidative stress on autophagocytotic degradation. Mech. Ageing. Dev., 1995, v. 81, i. 1, pp. 37−50.
- Katz M.L., EIdred G.E., Robison W.G. Lipofuscin autofluorescence: evidence for vitamin A involvement in the retina. Mech. Ageing. Dev., 1987, v. 39, i. 1, pp. 81−90.
- Abiko T., Abiko A., Ishiko S., Takeda M., Horiuchi S., Yoshida A. Relationship between auto fluorescence and advanced glycation end products in diabetic lenses. Exp. Eye Res., 1999, v. 68, i. 3, pp. 361−366.
- Sady C., Khosrof S., Nagaraj R. Advanced Maillard reaction and crosslinking of corneal collagen in diabetes. Biochem. Biophys. Res. Commun., 1995, v. 214, i. 3, pp. 793−797.
- Sell D.R., Lapolla A., Odetti P., Fogarty J., Monnier V.M. Pentosidine formation in skin correlates with severity of complications in individuals with long-standing IDDM. Diabetes, 1992, v. 41, i. 10, pp. 1286−1292.
- Bissonnette R., Zeng H., McLcan D.I., Schreiber W.E., Roscoe D.L., Lui H. Psoriatic plaques exhibit red autofluorescence that is due to protoporphyrin IX. J. Invest. Dermatol., 1998, v. Ill, i. 4, pp. 586−591.
- He X.Q., Li S.W., Hu Y.S., Lin J.X. Microspectrofluorometric analysis of autofluorescence in the cell walls of Phyllostachys pubescens culm. Acta Bot. Sin., 1999, v. 41, pp. 711−714.
- Donaldson L.A., Singh A.P., Yoshinaga A., Can K.T. Lignin distribution in mild compression wood of Pinus radiate. J. Bot., 1999, v. 77, i. 1, pp. 41−50.
- Gunning B.E.S., Schwartz O. Confocal microscopy of thylakoid autofluorescence in relation to origin of grana and phylogeny in the green algae. J. Plant Physiol., 1999, v. 26, pp. 695−708.
- Shaner N., Steinbach P., Tsien R.Y. A guide to choosing fluorescent proteins. Nature Methods, 2005, v. 2, № 12, pp. 905−909.
- Roda A., Guardigli M., Michelini E., Mirasoli M. Nanobioanalytical luminescence: Forster-type energy transfer methods. Anal. Bioanal. Chem., 2009, v. 393, pp. 109−123.
- Van de Lest C.H., Versteeg E.M., Veerkamp J.H., Van Kuppevelt T.H. Elimination of Autofluorescence in Immunofluorescence Microscopy with Digital Image Processing. J. Histochem. Cytochem., 1995, v. 43, i. 7, pp. 727−730.
- Terpetschnig E., Szmacinski H., Malak H., Lakowicz J.R. Metal-Ligand Complexes as a New Class of Long-Lived Fluorophores for Protein Hydrodynamics. Biophys. J., 1995, v. 68, pp. 342−350.
- Piszczek G. Luminescent metal-ligand complexes as probes of macromolecular interactions and biopolymer dynamics. Arch. Biochem. Biophys., 2006, v. 453, № 1, pp. 54−62.
- Thibon A., Pierre V.C. Principles of lanthanide-based luminescent probes for cellular imaging. Anal. Bioanal. Chem., 2009, v. 394, pp. 107−120.
- Rajapakse H.E., Reddy D. R,. Mohandessi S., Butlin N.G., Miller L.W. Luminescent terbium protein labels for time-resolved microscopy and screening. Angew. Chem. Int. Ed. Engl., 2009, v. 48, i. 27, pp. 4990−4992.
- Lissemore J.L., Jankowski J.T., Thomas C.B., Mascotti D.P., deHaseth P.L. Green fluorescent protein as a quantitative reporter of relative promoter activity in E. coli. -Biotechniques., 2000, v. 28, i. 1, pp. 82−84.
- Galland P., Senger H. New trends in photobiology the role of flavins as photoreceptors. J. Photochem. Photobiol. B: Biol., 1988, v. 1, pp. 277−294.
- Cowen T., Haven A.J., Burnstock G. Pontamine sky blue: a counterstain for background autofluorescence in fluorescence and immunofluorescence histochemistry. Histochem., 1985, v. 82, i. 3, pp. 205−208.
- Neumann M., Gabel D. Simple Method for Reduction of Autofluorescence in Fluorescence Microscopy. J. Histochem. Cytochem., 2002, v. 50, i. 3, pp. 437−439.
- Miiller-Taubenberger A., Anderson K.I. Recent advances using green and red fluorescent protein variants. Appl. Microbiol. Biotechnol., 2007, v. 77, i. 1, pp. 1−12.
- Binnemans K. Lanthanide-Based Luminescent Hybrid Materials. Chem. Rev., 2009, v. 109, pp. 4283−4374.
- Reisfeld R. Rare earth comlpexes in sol-gel glasses. Materials Science, 2002, v. 20, № 2, pp. 5−18.
- Motson G., Fleming J., Brooker S. Potential applications for the use of lanthanide complexes as luminescent biolabels. Advan. Inorg. Chem., 2004,. v. 55, pp. 361−431.
- Brunet E., Juanes O., Rodriguez-Ubis J.C. Supramolecularly Organized Lanthanide Complexes for Efficient Metal Excitation and Luminescence as Sensors in Organic and Biological Applications. Curr. Chem. Biol., 2007, v. 1, pp. 11−39.
- Biinzli J.C. Luminescent Lanthanide Probes as Diagnostic and Therapeutic Tools. Met. Ions Biol. Syst, 2004, v. 42, pp. 39−75.84.http://las.perkinelmer.com
- Lobnik A., Majeen N., Niederkiter K., Uray G. Optical pH sensor based on the absorption of antenna generated europium luminescence by bromothymolblue in a sol-gel membrane. Sens. Actuators, 2001, v. 74, pp. 200−206.
- Brunet E., Juanes O., Rodriguez-Ubis J.С. Supramolecularly Organized Lanthanide Complexes for Efficient Metal Excitation and Luminescence as Sensors in Organic and Biological Applications. Curr. Chem. Biol., 2007, v. 1, pp. 11−39.
- Berlman I.B. Energy transfer parameters of aromatic compounds. N.Y.: Academic Press, 1973, pp. 70−80.
- Mathis G. Rare earth cryptates and homogeneous fluoroimmunoassays with human sera. -Clin. Chem., 1993, v. 39, pp. 1953−1959.
- Selvin P.R. Principles and biophysical applications of lanthanide-based probes. Annu. Rev. Biophys. Biomembr., 2002, v. 31, pp. 275−302.
- Weissman S.I. Intramolecular Energy Transfer The Fluorescence of Complexes of Europium. J. Chem. Phys., 1942, v. 10, pp. 214−217.
- Balzani V. Photochemistry and luminescence of coordination compounds. J. Photochem. Photobiol. A: Chemistry, 1990, v. 51, p. 55−62.
- Whan R.E., Crosby G.A. Luminescence studies of rare earth complexes: Benzoylacetonate and dibenzoylmethide chelates. J. Mol. Spectrosc., 1962, v. 8, ii. 1−6, pp. 315−327.
- Crosby G.A., Whan R.E., Alire R.M. Intramolecular Energy Transfer in Rare Earth Chelates. Role of the Triplet State. J. Chem. Phys., 1961, v. 34, pp. 743−748.
- Crosby G.A., Whan R.E., Freeman J.J. Spectroscopic studies of rare earth chelates. J. Phys. Chem., 1962, v. 66, pp. 2493−2499.
- Misra V., Mishra H. Photoinduced proton transfer coupled with energy transfer: Mechanism of sensitized luminescence of terbium ion by salicylic acid doped in polymer. J. Chem. Phys., 2008, v. 128, i. 24, pp. 244 701.
- Breen P.J., Hild E.K., Horrocks W.D.Jr. Spectroscopic studies of metal ion binding to a tryptophan-containing parvalbumin. Biochem. J., 1985, v. 24, i. 19, pp. 4991−4997.
- Supkowski R.M., Bolender J.P., Smith W.D., Renyolds L.E.L., Horrocks W.D.Jr. Lanthanide Ions as Redox Probes of Long-Range Electron Transfer in Proteins. Coord. Chem. Rev., 1999, v. 185, pp. 307−319.
- Lazarides Т., Sykes D., Faulkner S., Barbieri A., Ward M.D. On the Mechanism of d-f Energy Transfer in RuII/Lnlll and OsII/Lnlll Dyads: Dexter-Type Energy Transfer Over a Distance of 20 A. Chem. Eur. J., 2008, v. 14, pp. 9389−9399.
- Мешкова С.Б., Кирияк А. В., Топилова З. М., Левшов С. М. Способы повышения чувствительности люминесцентного определения лантанидов с использованием их комлпексных соединений. Вюник Харктвського нацюнального ушверситету, 2008, вип. 16, № 39, с. 59−75.
- Eliseeva S.V., Bunzli J.-C.G. Lanthanide luminescence for functional materials and biosciences. Chem. Soc. Rev., 2010, v. 39, pp. 189−227.
- Mukkala V.M., Takalo H., Liitti P., Kankare J., Kuusela S., Lonnberg H. Lanthanide chelates as a tool in nucleic Acid chemistry. Met. Based. Drugs, 1994, v. 1, ii. 2−3, pp. 201 211.
- Pandya S., Yu J., Parker D. Engineering emissive europium and terbium complexes for molecular imaging and sensing. Dalton Trans., 2006, pp. 2757−2766.
- Savitsky A.P., Chydinov A.V., Krilova, S. M. 1995. Novel Fluorescent Chelate for Eu. Presented at the Advances in Fluorescence Sensing Technology II, San Jose С A 1995
- Р-Дикетонаты металлов. M.: Наука, 1980, 217 с.
- Va’zquez-Ibar J.L., Weinglass A.B., Kaback H.R. Engineering a terbium-binding site into an integral membrane protein for luminescence energy transfer. PNAS USA, 2002, v. 99, № 6, pp. 3487−3492.
- Cotton S. Lanthanides and Actinides. Oxford University Press., New York., 1991.111 vBunzli J-C.G. Benefiting from the unique properties of lanthanides ions. — Acc. Chem. Res., 2006, v. 39, i. l, pp. 53−61.
- MacManus J.P., Hogue C.W., Marsden B.J., Sikorska M., Szabo A.G. Terbium luminescence in synthetic peptide loops from calcium-binding proteins with different energy donors. J. Biol. Chem., 1990, v. 265, № 18, pp. 10 358−10 366.
- Franz K.J., Nitz M., Imperiali B. Lanthanide-binding tags as versatile protein coexpression probes. ChemBioChem., 2003, v. 4, pp. 265−271.
- Nitz M., Franz K.J., Maglathlin R.L., Imperiali B. Powerful combinatorial screen to identify high-affinity terbium (III)-binding peptides. ChemBioChem., 2003, v. 4, pp. 272−276.
- Martin L.J., Sculimbrene B.R., Nitz M., Imperiali B. Rapid Combinatorial Screening of Peptide Libraries for the Selection of Lanthanide-Binding Tags (LBTs). QSAR Comb. Sei., 2005, № 10, pp. 1149−1157.
- Nitz, M.- Sherawat, M.- Franz, K.J.- Peisach, E- Allen, K.N.- Imperiali, B. Structural origin of the high affinity of a chemically evolved lanthanide-binding peptide. Angew. Chem. Int. Ed. 2004,43,3682−3685.
- Porath J., Carlsson J., Olsson I., Beifrage G. Metal chelate affinity chromatography, a new approach to protein fractionation. Nature, 1975, v. 258, pp. 598−599.
- Sculimbrene B.R., Imperiali B. Lanthanide-Binding Tags as Luminescent Probes for Studying Protein Interactions. J. Am. Chem. Soc., 2006, v. 128, i. 22, pp. 7346−7352.
- Reynolds A.M., Sculimbrene B.R., Imperiali B. Lanthanide-Binding Tags with Unnatural Amino Acids: Sensitizing Tb3+ and Eu3+ Luminescence at Longer Wavelengths. Bioconjugate Chem., 2008, v. 19, pp. 588−591.
- Martin L.J., Hahnke M.J., Nitz M., Wohnert J., Silvaggi N.R., Allen K.N., Schwalbe H., Imperiali B. Double-lanthanide-binding tags: design, photophysical properties, and NMR applications. J. Am. Chem. Soc., 2007, v. 129, i. 22, pp. 7106−7113.
- Tyrell R.M., Keyse S.M. The interaction of UVA radiation with cultured cells. J. Photochem. Photobiol., 1990, v. 4, pp. 349−361.
- Kaiser W., Garrett C.G.B. Two-Photon Excitation in CaF2: Eu2. Phys. Rev. Lett., 1961, v. 7, i. 6, pp. 229−231.
- Singh S., Bradley L.T. Three-Photon Absorption in Naphthalene Crystals by Laser Excitation. Phys. Rev. Lett., 1964, v. 12, pp. 612−614.
- Lim E.C. Excitcd states. N.Y.: Academic Press, 1977, pp 1−56.
- Kliger D. Ultrasensitive laser spectroscopy. -N.Y.: Academic Press, 1983, pp. 109−174.
- Shreve A.P., Trautman J.K., Owens T.G., Albrecht A.C. Two-photon excitation spectroscopy of thylakoid membranes from Phaeodactylum tricornutum: Evidence for an in vivo two-photon allowed carotenoid state. Chem. Phys. Lett., 1990, v. 170, pp. 51−56.
- Pantell R. H., Pradere F., Hanus J., Schott M., Puthoff H. Theoretical and Experimental Values for Two, Three and Four Photon Absorption. J. Chem. Phys., 1967, v. 46, pp. 35 073 511.
- Shreve A.P., Albrecht A.C. A three-photon fluorescence excitation study of the SO (Alg) to Sl (B2u) transition in neat liquid benzene. J. Chem. Phys., 1991, v. 94, pp. 5772−5773.
- Denk W., Strickler J.H., Webb W.W. Two-Photon Laser Scanning Fluorescence Microscopy. Science, 1990, v. 248, № 4951, pp. 73−76.
- Diaspro A., Bianchini P., Vicidomini G., Faretta M., Ramoino P., Usai C. Multi-photon excitation microscopy. Biomed. Eng Online, 2006, v. 5:36.
- Guild J.B., Xu C., Webb W.W. Measurement of group velocity dispersion of high numerical aperture objective lenses using two-photon excited fluorescence. Appl. Opt., 1997, v. 36, pp. 397−401.
- Konig K., So P.T.C., Mantulin W.W., Gratton E. Cellular response to near-infrared femtosecond laser pulses in two-photon microscopes. Opt. Lett., 1997, v. 22, pp. 135−136.
- Konig K., So P.T.C., Mantulin W.W., Tromberg B.J., Gratton E. Two-photon excited lifetime imaging of autofluorescence in cells during UVA and NIR photostress. J. Micros., 1996, v. 183, pp. 197−204.
- Dunn K.W., Young P.A. Principles of Multiphoton Microscopy. Nephron Exp. Nephrol., 2006, v. 103, № 2, pp. e33−40.
- Koenig K. Multiphoton microscopy in life sciences. J. Microsc., 2000, v. 200, i. 2, pp. 83 104.
- Tauer U. Advantages and risks of multiphoton microscopy in physiology. Exp Physiol., 2002, v. 87, № 6, pp. 709−714.
- Oheim M., Beaurepaire E., Chaigneau E., Mertz J., Charpak S. Two-photon microscopy in brain tissue: parameters influencing the imaging depth. J. Neurosci. Methods, 2001, v. 111, pp. 29−37.
- Zipfel W.R., Williams R.M., Webb W.W. Nonlinear magic: multiphoton microscopy in the biosciences. Nat. Biotechnol., 2003, v. 21, pp. 1369−1377.
- Kim H. M., Cho B. R. Two-Photon Probes for Intracellular Free Metal Ions, Acidic Vesicles, And Lipid Rafts in Live Tissues. Acc. Chem. Res., 2009, v. 42, pp. 863−872.
- Masters B.R., So P.T.C. Antecedents of Two-Photon Excitation Laser Scanning Microscopy. Microscopy Research and Technique, 2004, v. 63, pp. 3−11.
- Lakowicz J.R., Piszczek G., Maliwal B.P., Gryczynski I. Multiphoton excitation of lanthanides. ChemPhysChem., 2001, v. 2, pp. 247−252.
- Piszczek G., Maliwal B.P., Gryczynski I., Dattelbaum J., Lakowicz J.R. Multiphoton Ligand-Enhanced Excitation of Lanthanides. J. Fluoresc., 2001, v. 11, № 2, pp. 101−107.
- White G.F., Litvinenko K.L., Meech S.R., Andrew D.L., Thompson AJ. Multiphoton-excited luminescence of a lanthanide ion in a protein complex: Tb bound to transferring. -Photochem. Photobiol. Sci., 2004, v. 3, pp. 47−55.
- Luo L" Lai W.P.-W., Wong K.-L., Wong W.-T., Li K.-F., Cheah K.-W. Green upconversion fluorescence in terbium coordination complexes. Chem. Phys. Lett., 2004, v. 398, pp. 372−376.
- Wong K.L., Law G.L., Kwok W.M., Wong W.T., Phillips D.L. Simultaneous observation of green multiphoton upconversion and red and blue NLO processes from polymeric terbium (III) complexes. Angew. Chem. Int. Ed. Engl., 2005, v. 44, i. 22, pp. 3436−3439.
- Law G.L., Wong K.L., Yang Y.Y., Yang H.L., Wong W. T,. Lam M.H., Tam H.L., Cheah K.W. Molecular switching in the near infrared (NIR) to visible/NIR f-f emission with a functional-lanthanide complexes. J. Fluoresc., 2008, v. 18, ii. 3−4, pp. 749−752.
- Law G.L., Kwok W.M., Wong W.T., Wong K.L., Tanner P.A. Terbium luminescence sensitized through three-photon excitation in a self-assembled unlinked antenna. J. Phys. Chem. B., 2007, v. lll, i. 37, pp. 10 858−10 861.
- Palsson L.O., Pal R., Murray B.S., Parker D., Beeby A. Two-photon absorption and photoluminescence of europium based emissive probes for bioactive systems. Dalton Trans., 2007, v. 48, pp. 5726−5734.
- D’Aleo A., Pompidor G., Elena B.5 Vicat J., Baldeck P.L., Toupet L., Kahn R., Andraud C., Maury O. Two-photon microscopy and spectroscopy of lanthanide bioprobes. Chemphyschem., 2007, v. 8, i. 14, pp. 2125−2132.
- Law G. L, Wong K. L, Man C.W.Y, Wong W. T, Tsao S. W, Lam M.H.W, Lam P.K.S. Emissive terbium probe for multiphoton in vitro cell imaging. J. Am. Chem. Soc., 2008, v. 130, i. 12, pp. 3714−3715.
- Selvin P.R. The renaissance of fluorescence resonance energy transfer. Nat. Struct. Biol., 2000, v. 7, № 9, pp. 730−734.
- Selvin P.R. Fluorescence Resonance Energy Transfer. Methods Enzymol., 1995, v. 246, pp. 300−334.
- Giordano L., Jovin T.M., Irie M., Jares-Erijman E.A. Diheteroarylethenes as Thermally Stable Photoswitchable Acceptors in Photochromic Fluorescence Resonance Energy Transfer (pcFRET). J. Am. Chem. Soc., 2002, v. 124, № 25, pp. 7481−7489.112
- Yun С., You J., Kim J., Huh J., Kim E. Photochromic fluorescence switching from diarylethenes and its applications. Journal of Photochemistry and Photobiology C: Photochemistry Reviews, 2009, v. 10, i. 3, pp. 111−129.
- Ефремов E.C., Филатова М. П., Реутова Т. О., Степанова JI.H., Рейссман 3., Иванов В. Т. Конформационные состояния брадикинина и его аналогов в растворах, II. спектры флуоресценции. Биоорганическая химия, 1977, т. 3, № 9, с. 1169−1180.
- Kohen Е., Hirschberg J.G., Ploem J.S. FRET microscopy: Digital imaging of fluorescence resonance energy transfer. Application in cell biology. In: Cell Structure and Function by Micro spectra fluometry. London: Academic Press, 1989, pp. 99−117.
- Madan R., Satyajit M. Use of Forster’s resonance energy transfer microscopy to study lipid rafts. Biochimica et Biophysica Acta, 2005, pp. 221−233.
- Torres Т., Levitus M. Measuring conformational dynamics: A new FCS-FRET approach. -J. Phys. Chem., 2007, v. 111, № 25, pp. 7392−7400.
- Olwin B.B., Keller C.H., Storm D.R. Interaction of a fluorescent N-dansylaziridine derivative of troponin I with calmodulin in the absence and presence of calcium. Biochemistry, 1982, v. 21, pp. 5669−5675.
- Chapman, E.R., Alexander K., Vorherr Т., Carafoli E., Storm D.R. Fluorescence energy transfer analysis of calmodulin-peptide complexes. Biochemistry, 1992, v. 31, pp. 12 819— 12 825.
- Shotten D.M. Confocal scanning optical microscopy and its applications for biological specimens. J. Cell Sci., 1989, v. 94, pp. 175−206.
- Gordon G.W., Berry G., Liang X. H., Levine В., Herman B. Quantitative fluorescence resonance energy transfer measurements using fluorescence microscopy. Biophys. J., 1998, v. 74, pp. 2702−2713.
- Giordano L., Vermeij R.J., Jares-Erijman E.A. Synthesis of indole-containing diheteroarylethenes. New probes for photochromic FRET (pcFRET). ARKIVOC, 2005, v. 12, pp. 268−281.
- Song L., Jares-Erijman E.A., Jovin T.M. A photochromic acceptor as a reversible light-driven switch in fluorescence resonance energy transfer (FRET). J. Photochem. Photobiol. A: Chem., 2002, v. 150, ii. 1−3, № 26, pp. 177−185.
- Schiller P.W. Study of Adrenocorticotropic Hormone Conformation by Evaluation of Intramolecular Resonance Energy Transfer in N-Dansyllysine21- ACTH-(l-24)-Tetrakosipeptide. PNAS USA, 1972, v. 69, № 4, pp. 975−979.
- Miller J.N. Fluorescence energy transfer methods in Bioanalysis Analyst, 2005, v. 130, pp. 265−270.
- Berney C., Danuser G. FRET or no FRET: a quantitative comparison. Biophysical J., 2003, v. 84, pp. 3992−4010.
- Van Munster E.B., Gadella T.W. Fluorescence lifetime imaging microscopy (FLIM). Adv. Biochem. Eng. Biotechnol, 2005, v. 95, pp. 143−175.
- Wallrabe H., Periasamy A. Imaging protein molecules using FRET and FLIM microscopy. -Curr. Opin. Biotechnol., 2005, v. 16, pp. 19−27.
- Remaut K., Lucas В., Braeckmans K., Sanders N.N., De Smedt S.C., Demeester J. FRET-FCS as a tool to evaluate the stability of oligonucleotide drugs after intracellular delivery. J. Control Release, 2005, v. 103, i. 1, pp. 259−271.
- Wallace M.I., Ying L., Balasubramanian S., Klenerman D. FRET fluctuation spectroscopy: exploring the conformational dynamics of a DNA hairpin loop. J. Phys. Chem. B, 2000, v. 104, № 48, pp. 11 551−11 555.
- Eggeling C., Jager S., Winkler D., Kask P. Comparison of different fluorescence fluctuation methods for their use in FRET assays: monitoring a protease reaction. Curr. Pharm. Biotechnol., 2005, v. 6, i. 5, pp. 351−371.
- Gratton E., Breusegem S., Barry N., Ruan Q., Eid J. Fluctuation Correlation Spectroscopy in Cells: Determination of molecular aggregation. Biophotonics, 2005, pp. 1−14.
- Mann T.L., Krull U.J. Fluorescence polarization spectroscopy in protein analysis. -Analyst., 2003, v. 128, pp. 313−317.
- Mattheyses A.L., Hoppey A.D., Axelrod D. Polarized Fluorescence Resonance Energy Transfer Microscopy. Biophys. J., 2004, v. 87, pp. 2787−2797.
- Levitt J.A., Matthews D.R., Ameer-Beg S.M., Suhling K. Fluorescence lifetime and polarization-resolved imaging in cell biology. Curr Opin Biotechnol., 2009, v. 20, № 1, pp. 2836.
- Gautier I., Tramier M., Durieux C., Coppey J., Pansu R. В., Nicolas J. C., Kemnitz K., Coppey-Moisan M. Homo-FRET microscopy in living cells to measure monomer-dimer transition of GFP-tagged proteins. Biophys. J., 2001, v. 80, pp. 3000−3008.
- Runnels L.W., Scarlata S.F. Theory and application of fluorescence homotransfer to melittin oligomerization. Biophys. J., 1995, v. 69, pp. 1569−1583.
- Tramier M, Coppey-Moisan M. Fluorescence anisotropy imaging microscopy for homo-FRET in living cells. Methods Cell Biol., 2008, v. 85, pp. 395−414.
- Rizzo M.A., Piston D.W. High-contrast imaging of fluorescent protein FRET by fluorescence polarization microscopy. Biophys. J., 2005, v. 88, pp. L14-L16.
- Matsuya Т., Hoshino N., Okuyama T. Curr. Anal. Chem., 2006, 2, i. 4, pp. 397−410.
- T. Nishioka, K. Fukui and K. Matsumoto, in Handbook on the Physics and Chemistry of Rare Earths, ed. K. A. Gschneidner, Jr, J.-C. G. Bu. nzli and V. K. Pecharsky, Elsevier Science B.V., Amsterdam, 2007, vol. 37.
- Spangler C.M., Spangler C., Schaerling M. Luminescent Lanthanide Complexes as Probes for the Determination of Enzyme Activities. Ann. N. Y. Acad. Sci., 2008, v. 1130, pp. 138−148.
- Bunzli J.-C.G. Lanthanide Luminescent Bioprobes (LLBs). Chem. Lett., 2009, v. 38, pp. 104−108.
- Hovinen J., Guy P.M. Bioconjugation with Stable Luminescent Lanthanide (III) Chelates Comprising Pyridine Subunits. Bioconjug Chem., 2008, v. 20, i. 3, pp. 404−421.
- Connally R.E., Piper J.A. Time-gated luminescence microscopy. Ann. N. Y. Acad. Sci., 2008, v. 1130, pp. 106−116.
- Vereb G., Jares-Erijman E., Selvin P.R., Jovin T.M. Temporally and spectrally resolved imaging microscopy of lanthanide chelates. Biophys. J., 1998, v. 74, i. 5, pp. 2210−2222.
- Bunzli J.-C.G., Chauvin A.-S., Vandevyver C.D.B., Song В., Comby S. Lanthanide Bimetallic Helicates for in Vitro Imaging and Sensing. Ann. N. Y. Acad. Sci., 2008, v. 1130, pp. 97−105.
- Montgomery C.P., Murray B.S., New E.J., Pal R., Parker D. Cell-penetrating metal complex optical probes: targeted and responsive systems based on lanthanide luminescence. — Acc. Chem. Res., 2009, v. 42, i. 7, pp. 925−937.
- Song В., Wang G., Tan M., Yuan J. A Europium (ln) Complex as an Efficient Singlet Oxygen Luminescence Probe. J. Am. Chem. Soc., 2006, v. 128, pp. 13 442−14 450.
- Soini E., Hemmila I. Fluoroimmunoassay-present status and key problems. Clin. Chem., 1979, v. 25, i. 3, pp. 353−361.
- Hemmila I., Mukkala V.M. Time-resolution in fluorometry technologies, labels, and applications in bioanalytical assays. Crit. Rev. Clin. Lab. Sci., 2001, v. 38, i. 6, pp. 441−519.
- Steinkamp Т., Karst U. Detection strategies for bioassays based on luminescent lanthanide complexes and signal amplification. Anal. Bioanal. Chem., 2004, v. 380, № 1, pp. 24−30.
- Vazquez-Ibar J.L., Weinglass A.B., Kaback H.R. Engineering a terbium-binding site into an integral membrane protein for luminescence energy transfer. PNAS USA, 2002, v. 99, i. 6, pp. 3487−3492.
- Rajapakse H.E., Reddy D.R., Mohandessi S., Butlin N.G., Miller L.W. Luminescent Terbium Protein Labels for Time-Resolved Microscopy and Screening. Angew. Chem. Int. Ed., 2009, v. 48, pp. 4990 -4992.
- Савицкий А.П., Папковский Д. Б., Пономарев T.B., Березин И. В. Фосфоресцентный иммуноанализ. Металлопорфирины альтернатива редкоземельным флуоресцентным меткам. — Докл. АН СССС, 1989, т.304, № 4, с.1005−1008.
- Савицкий А.П., Папковский Д. Б., Флуоресцентный иммуноанализ. Порфирины -новый тип меток для иммуноанализа. Докл. АН СССР, 1987, т. 293, вып. 3, с. 744−745.
- Kronick M.N. The use of phycobiliproteins as fluorescent labelsin immunoassays. J. Immunol. Methods, 1986, v. 92, pp.1−13.
- Miller J.N. Fluorescence energy transfer methods in bioanalysis. Analyst, 2005, v. 130, pp. 265−270.
- Yeh S.W., Ong L.J., Glazer A.N., Clark J.H. Fluorescence properties of allophycocyanin and a crosslinked allophycocyanin trimer. Cytometry, 1987, v. 8, pp. 91−95.
- Degorce F., Card A., Soh S., Trinquet E., Knapik G.P., Xie B. HTRF: A Technology Tailored for Drug Discovery -A Review of Theoretical Aspects and Recent Applications. Curr. Chem. Genomics, 2009, v. 3, pp. 22−32.
- Kupcho K.R., Stafslien D.K., DeRosier T., Hallis T.M., Ozers M.S., Vogel K.W. Simultaneous Monitoring of Discrete Binding Events Using Dual-Acceptor Terbium-Based LRET. J. Am. Chem. Soc., 2007, v. 129, i. 44, pp 13 372−13 373.
- Kokko T., Kokko L., Soukka T. Terbium (III) Chelate as an Efficient Donor for Multiple-Wavelength Fluorescent Acceptors. J. Fluoresc., 2009, v. 19, pp. 159−164.
- Zhong W. Nanomaterials in fluorescence-based biosensing. Anal. Bioanal. Chem., 2009, v. 394, pp. 47−59.
- Sandtner W., Bezanilla F., Correa A.M. In Vivo Measurement of Intramolecular Distances Using Genetically Encoded Reporters. Biophys. J., 2007, v. 93, i. 9, pp. 45−47.
- Shimomura O., Johnson F. H., Saiga Y. Extraction, purification and properties of aequorin, a bioluminescent protein from the luminous hydromedusan, Aequorea. J. Cell. Comp. Physiol., 1962, v. 59, pp. 223−239.
- Prasher D.C., Eckenrode V.K., Ward W.W., Prendergast F.G., Cormier M.J. Primary structure of the Aequorea victoria green-fluorescent protein. Gene, 1992, v. 111, pp. 229−233.
- Heim R., Prasher D.C., Tsien R.Y. Wavelength mutations and posttranslational autoxidation of green fluorescent protein. PNAS USA, 1994, v. 91, pp. 12 501−12 504.
- Delagrade S., Hawtin R.E., Silva C.M., Yang M.M., Youvan D.C. Red-shifted excitation mutants of the green fluorescent protein. Biotechnology, 1995, v. 13, pp. 151−154.
- Ormy M., Cubitt A.B., Kallio K., Gross L.A., Tsien R.Y., Remington S.J. Crystal structure of the Aequorea victoria green fluorescent protein. Science, 1996, v. 273, pp. 1392−1395.
- Tsien R.Y. The green fluorescent protein. Annu. Rev. Biochem., 1998, v. 67, pp. 509−544.
- Matz M.V., Fradkov A.F., Labas Y.A., Savitsky A.P., Zaraisky A.G., Markelov M.L., Lukyanov S.A. Fluorescent proteins from nonbioluminescent Anthozoa species. Nat. Biotechnol., 1999, v. 17, pp. 969−973.
- Fradkov A.F., Chen Y., Ding L., Barsova E.V., Matz M.V., Lukyanov S.A. Novel fluorescent protein from Discosoma coral and its mutants possesses a unique far-red fluorescence. FEBS Lett., 2000, v. 479, pp. 127−130.
- Labas Y.A., Gurskaya N.G., Yanushevich Y.G., Fradkov A.F., Lukyanov K.A., Lukyanov S.A., Matz M.V. Diversity and evolution of the green fluorescent protein family. Proc. Nat. Acad. Sci. USA, 2002, v. 99, pp. 4256−4261.
- Морозова E.C., Верхуша В. В., Перский Е. Э. Флуоресцентные белки красной спектральной области. Вюник Харшвського нащонального ушверситету iMem В. Н. Каразша, 2009, вип. 9, № 856.
- Зубова Н., Савицкий А. П. Молекулярные клеточные сенсоры, созданные на основе цветных флуоресцирующих белков. Успехи биологической химии, 2005, т. 45, с. 391 454.
- VanEngelenburg В., Palmer А.Е. Fluorescent biosensors of protein function. Curr. Opin. Chem. Biol., 2008, v. 12, pp. 1−6.
- Tainaka K., Sakaguchi R., Hayashi H., Nakano S., Liew F.F., Morii T. Design Strategies of Fluorescent Biosensors Based on Biological Macromolecular Receptors. Sensors, 2010, v. 10, pp.1355−1376.
- Morris M.C. Fluorescent Biosensors of Intracellular Targets from Genetically Encoded Reporters to Modular Polypeptide Probes. Cell Biochem. Biophys., 2010, v. 56, pp. 19−37.
- Remington S.J. Fluorescent proteins: maturation, photochemistry and photophysics. Curr. Opin. Struct. Biol., 2006, v. 16, i. 6, pp. 714−721.118
- Morris M.C. Fluorescent Biosensors of Intracellular Targets from Genetically Encoded Reporters to Modular Polypeptide Probes. Cell Biochem. Biophys., 2010, v. 56, pp. 19−37.
- Zimmer M. Green Fluorescent Protein (GFP): Applications, Structure, and Related Photophysical Behavior. Chem. Rev., 2002, v. 102, i. 3, pp. 759−781.
- Ormo M, Cubitt A.B., Kallio K., Gross L.A., Tsien R.Y., Remington S.J. Crystal structure of the Aequorea Victoria green fluorescent protein. Science, 1996, v. 273, pp. 1392−1395.
- Yang F., Moss L.G., Phillips G.N.Jr. The molecular structure of green fluorescent protein. -Nat. Biotechnol., 1996, v. 14, pp. 1246−1251.
- Martin L-J. Development of LBTs as Powerfull and Versatile Peptides for use in Studies of Protein and Protein Interactions: doctoral thesis. Massachussets. 2008. 185 p.
- Matz M.V., Fradkov A.F., Labas Y.A., Savitsky A.P., Zaraisky A.G., Markelov M.L., Lukyanov S.A. Fluorescent proteins from nonbioluminescent Anthozoa species. Nat. Biotechnol., 1999, v. 17, pp. 969—973.
- Gross L.A., Baird G.S., Hoffman R.C., Baldridge K.K., Tsien R.Y. The structure of the chromophore within DsRed, a red fluorescent protein from coral. PNAS, 2000, v. 97, pp. 2 211 990−2 211 995.
- Wang Y., Shyy J.Y.-J., Chien S. Fluorescence Proteins, Live-Cell Imaging, and Mechanobiology: Seeing Is Believing. Annu. Rev. Biomed. Eng., 2008, v. 10, pp. 1−38.
- Miyawaki A. Green fluorescent protein-like proteins in reef Anthozoa animals. Cell Struct Funct. 2002, v. 5, pp. 343−347.245. http://www.pdb.org
- Strack R.L., Strongin D.E., Bhattacharyya D., Tao W., Berman A., Broxmeyer H.E., Keenan R.J., Glick B.S. A noncytotoxic DsRed variant for whole-cell labeling. Nat. Methods, 2008, v. 5, i. 11, pp. 955−957.
- Bevis B.J., Glick B.S. Rapidly maturing variants of the Discosoma red fluorescent protein (DsRed). Nat. Biotechnol., 2002, v. 20, pp. 83−87.
- Strack R.L., Strongin D.E., Bhattacharyya D., Tao W., Berman A., Broxmeyer H.E., Keenan R.J., Glick B.S. A noncytotoxic DsRed variant for whole-cell labeling. Nat. Methods, 2008, v. 5, i. 11, pp. 955−957.
- Kredel S., Oswald F., Nienhaus K., Deuschle K., Rocker C., Wolff M., Heilker R., Nienhaus G.U., Wiedenmann J. mRuby, a Bright Monomeric Red Fluorescent Protein for Labeling of Subcellular Structures. PLoS ONE, 2009, v. 4, i. 2, pp. 1−7.
- Sato Y., Igarashi Y., Hakamata Y., Murakami T., Kaneko T., Takahashi M., Seo N., Kobayashi E. Establishment of Alb-DsRed2 transgenic rat for liver regeneration research. -Biochem. Biophys. Res. Commun., 2003, v. 311, pp. 478−481.
- Yarbrough D., Wachter R.M., Kallio K., Matz M.V., Remington S.J. Refined crystal structure of DsRed, a red fluorescent protein from coral, at 2.0-A resolution. PNAS USA, 2001, v. 98, i. 2, pp. 462−467.
- Robers M.B., Machleidt T., Carlson C.B., Bi K. Cellular LanthaScreen and beta-lactamase reporter assays for high-throughput screening of JAK2 inhibitors. Assay Drug. Dev. Technol., 2008, v. 6, i. 4, pp. 519−529.
- Carlson C.B., Robers M.B., Vogel K.W., Machleidt T. Development of LanthaScreen cellular assays for key components within the PI3K/AKT/mTOR pathway. J. Biomol. Screen., 2009, v. 14, i. 2, pp. 121−132.
- Carlson C.B., Mashock M.J., Bi K. BacMam-enabled LanthaScreen cellular assays for PI3K/Akt pathway compound profiling in disease-relevant cell backgrounds. J. Biomol. Screen., 2010, v. 15, i. 3, pp. 327−334.
- Vuojola J., Lamminmaki U., Soukka T. Resonance energy transfer from lanthanide chelates to overlapping and nonoverlapping fluorescent protein acceptors. Anal. Chem., 2009, v. 81, i. 12, pp. 5033−5038.
- Laitala V., Hemmilla I. Homogeneous Assay Based on Anti-Stokes' Shift Time-Resolved Fluorescence Resonance Energy-Transfer Measurement. Anal. Chem., 2005, v. 77, i. 5, pp 1483−1487.
- Kokko L., Sanberg K., Lovgren T., Soukka T. Europium (III) chelate -dyed nanoparticles as donors in a homogeneous proximity-based immunoassay for estradiol. Anal. Chim. Acta, 2004, v. 503, pp. 155−162.
- Appelblom H., Nurmi J., Soukka T., Pasternack M., Penttila K.E., Lovgren T., Niemela P. Homogeneous TR-FRET High-Throughput Screening Assay for Calcium-Dependent Multimerization of Sorcin. J. Biomol. Screen., 2007, v. 12, № 6, pp. 842−848.
- Wang G., Yuan J., Hai X., Matsumoto K. Homogenous Time-resolved Fluroimmunoassay of 3,5,3-triiodo-L-thyronine in Human Serum by Using Europium Fluorescence Energy Transfer. Talanta, 2006, v. 70, i. 1, pp. 133−138.
- Heyduk T., Heyduk E. Luminescence Energy Transfer with Lanthanide Chelates: Interpretation of Sensitized Acceptor Decay Amplitudes. Anal. Biochem., 2001, v. 289, i. 1, pp. 60−67.
- Chen Y., Lehrer S.S. Distances between Tropomyosin Sites Across the Muscle Thin Filament Using Luminescence Resonance Energy Transfer: Evidence for Tropomyosin Flexibility. Biochemistry, 2004, v. 43, № 36, pp. 11 491−11 499.
- Sueda S., Yuan J., Matsumoto K. A homogeneous DNA hybridization system by using a new luminescence terbium chelate. Bioconjugate Chem., 2002, v. 13, pp. 200−205.
- Nurmi J., Wikman T., Karp M., Lovgren T. High-Performance Real-Time Quantitative RT-PCR Using Lanthanide Probes and a Dual-Temperature Hybridization Assay. Anal. Chem., 2002, v. 74, pp. 3525−3532.
- Mathis G. Probing molecular interactions with homogeneous techniques based on rare earth cryptates and fluorescence energy transfer. Clin. Chem., 1995, v. 41, pp. 1391−1397.
- Laitala V., Ylikoski, A., Raussi H.M., Ollikka P., Hemmila I. Time-resolved detection probe for homogeneous nucleic acid analyses in one-step format. — Anal. Biochem., 2007, v. 361, pp. 126−131.
- Guo W., Urizar E., Kralikova M., Mobarec J.C., Shi L., Filizola M., Javitch J.A. Dopamine D2 receptors form higher order oligomers at physiological expression levels. EMBO J., 2008, v. 27, № 17, pp. 2293−2304.
- Wang J., Norcross M. Dimerization of chemokine receptors in living cells: key to receptor function and novel targets for therapy. Drug Discov. Today, 2008, v. 13, ii. 13−14, pp. 625−632.
- Dodeller F., Gottar M., Huesken D., Iourgenko V., Cenni B. The lysosomal transmembrane protein 9B regulates the activity of inflammatory signaling pathways. J. Biol. Chem., 2008, v. 283, № 31, pp. 21 487−21 494.
- Goedken E.R., Gagnon A.I., Overmeyer G.T., Liu J., Petrillo R.A., Burchat A.F., Tomlinson M.J. HTRF-based assay for microsomal prostaglandin E2 synthase-1 activity. J. Biomol. Screen., 2008, v. 13 № 7, pp. 619−625.
- Lopez-Crapez E., Bazin H., Andre E., Noletti J., Greinier J., Mathis G. A homogeneous europium cryptate-based assay for the diagnosis of mutations by time-resolved luminescence resonance energy transfer. Nucleic Acids Res., 2001, v. 29, p.1−10.
- Sambrook J., Fritisch E.F., Maniatis T. Molecular Cloning: a Laboratory Manual, 2nd Ed. -N.Y.: Cold Spring Harbor Laboratory Press, 1989.
- Yanisch-Perron C., Vieira, J, Messing J. Improved M13 phage cloning vectors and host strains: nucleotide sequences of the M13mpl8 and pUC19 vectors. Gene, 1985, v. 33, i. 1, pp. 103−119.
- Laemmli U.K. Cleavage of Structural Proteins during the Assembly of the Head of Bacteriophage T4. Nature (London), 1970, v. 227, pp. 680−685.
- Nitz M., Franz K.J., Maglathlin R.L., Imperial! B. A powerful combinatorial screen to identify high-affmity terbium (III)-binding peptides. Chembiochem., 2003, v. 4, pp. 272−276.
- Karasawa S., Araki Т., Nagai Т., Mizuno H., Miyawaki A. Cyan-emitting and orange-emitting fluorescent proteins as a donor/acceptor pair for fluorescence resonance energy transfer. Biochem. J., 2004, v. 381, i. 1, pp. 307−312.290. www.neb.com
- Subach F.V., Patterson G.H., Renz M., Lippincott-Schwartz J., Verkhusha V.V. Bright monomelic photoactivatable red fluorescent protein for two-color super-resolution sptPALM of live cells. J. Am. Chem. Soc., 2010, v. 132, pp. 6481−6491.
- Angres В., Steuer H., Weber P., Wagner M., Schneckenburger H. A Membrane-Bound FRET-Based Caspase Sensor for Detection of Apoptosis Using Fluorescence Lifetime and Total Internal Reflection Microscopy. Cytometry Part A, 2009, v. 75A, pp. 420−427.123
- Parker D., Williams J.A.G. The lanthanides and their interrelations with biosystems. Metal ions in biological systems, 2003, v. 40, pp. 262−264.
- Основы ферментативной кинетики. Корниш-Боуден Э. М: Мир, 1979.
- Mendoza-Alvarez H., Alvarez-Gonzalez R. Poly (ADP-ribose) polymerase is a catalytic dimer and the automodification reaction is intermolecular. J. Biol. Chem., 1993, v. 268, pp. 22 575−22 580.
- Lozanov, V., Ivanov, I.P., Benkova, В., and Mitev, V. (2009) Amino Acids, 36, 581−586.
- Lien, S., Pastor, R., Sutherlin, D., and Lowman, H.B. (2004) Protein J., 23, 413−425.
- Sun, J., Bottomley, S.P., Kumar, S., and Bird, P.I. (1997) Biochem. Biophys. Res. Commun., 238, 920−924.
- Garcia-Calvo, M., Peterson, E.P., Rasper, D.M., Vaillancourt, J.P., Zamboni, R., Nicholson, D.W., and Thornberry, N.A. (1999) Cell Death Differ., 6, 362−369.
- Chiang J.J.-H., Truong K. Computational Modeling of a New Fluorescent Biosensor for Caspase Proteolytic Activity Improves Dynamic Range. IEEE Trans. Nanobioscience, 2006, v. 5, № 1, pp. 41−45.
- Tawa P., Tam J., Cassady R., Nicholson D.W., Xanthoudakis S. Quantitative analysis of fluorescent caspase substrate cleavage in intact cells and identification of novel inhibitors of apoptosis. Cell Death Differ., 2001, v. 8, pp. 30−37.
- Lin J., Zhang Z., Yang J., Zeng S., Liu B.F., Luo Q. Real-time detection of caspase-2 activation in a single living HeLa cell during cisplatin-induced apoptosis. J. Biomed. Opt., 2006, v. 11, i. 2, 24 011.